Inclusões, Engenharia de Inclusão e Aços Limpos
Inclusões, Engenharia de Inclusão e Aços Limpos
As inclusões são compostos não metálicos e precipitados que se formam no aço durante sua produção e processamento e, portanto, são os subprodutos da fabricação de aço que surgem de diferentes químicas e processos. As inclusões podem variar muito em tamanho e composição, dando origem a uma ampla gama de efeitos correspondentes e exigindo equipamentos analíticos sofisticados para caracterização.
As inclusões são constituídas por fases vitrocerâmicas embutidas em matriz metálica de aço. O controle de inclusão é promover a remoção de inclusões do aço e reduzir seus efeitos nocivos na qualidade e no processamento do aço. É um aspecto importante da prática siderúrgica. No entanto, a presença de certos tipos de inclusão também pode produzir efeitos benéficos no aço.
A origem, a remoção e as consequências mecânicas das inclusões dependem de seus tipos e de sua engenharia. A composição química das inclusões e sua fração volumétrica são determinadas pelo gerenciamento das diferentes etapas envolvidas no processo de produção, como as operações de fusão, refino e fundição. Assim, a população de inclusão depende da relação existente entre os parâmetros operativos aplicados e as características dos aços a serem produzidos.
A evolução de uma população de inclusão na panela é influenciada por vários fatores, como o tipo e a distribuição de tamanho das inclusões de origem no forno de aciaria na extração, o nível de oxigênio na extração, a quantidade de escória transportada do forno de aciaria, o tipo, quantidade e tempo de adição de escória sintética à panela, o tipo e tempo de adição de desoxidante e o tempo e a intensidade de agitação na panela para citar alguns. A forma das inclusões pode ser globular, plaquetária, dendrítica ou poliédrica.
A forma globular é desejável. Certas inclusões como MnS (sulfeto de manganês), oxi-sulfetos formados durante a solidificação nos espaços entre os braços dendritos, aluminatos e silicatos de ferro são globulares. A forma das plaquetas é indesejável. Os aços desoxidados com alumínio contêm MnS na forma de filmes finos localizados ao longo dos contornos de grão. Inclusões poliédricas não são muito prejudiciais. As inclusões têm formas diferentes que são descritas abaixo. A forma globular das inclusões é a mais desejada, pois seu efeito nas propriedades mecânicas do aço é moderado. A forma esférica de inclusões globulares é o resultado de sua formação em estado líquido com baixo teor de alumínio.
Inclusões em forma de plaquetas existem em aços que são desoxidados pelo alumínio. Essas inclusões contêm MnS e oxi-sulfetos na forma de filmes finos (plaquetas) localizados ao longo dos contornos de grão do aço. Tais inclusões são formadas como resultado da transformação eutética durante a solidificação. Inclusões em forma de plaquetas não são desejáveis. Eles enfraquecem consideravelmente os contornos de grão e causam efeitos adversos nas propriedades mecânicas, particularmente em condições quentes (curtência a quente).
As inclusões em forma de dendrito são devidas ao uso de quantidade excessiva de desoxidante forte (alumínio). Isso resulta na formação de inclusões de óxido e sulfeto em forma de dendrito (separadas e agregadas). Essas inclusões possuem ponto de fusão maior que o do aço. Arestas vivas e cantos das inclusões em forma de dendrito podem causar concentração local de tensão interna, o que diminui consideravelmente a ductilidade, tenacidade e resistência à fadiga do aço.
As inclusões poliédricas são formadas quando a morfologia das inclusões em forma de dendrito é melhorada pela adição (após desoxidação profunda pelo alumínio) de pequenas quantidades de elementos de terras raras (cério, lantânio) ou alcalino-terrosos (cálcio, magnésio). Uma vez que sua forma se aproxima da forma globular, as inclusões poliédricas causam menos efeito nas propriedades do aço do que as inclusões em forma dendrítica.
Existem microinclusões (tamanho de 1 micrômetro a 100 micrômetros) e macroinclusões (tamanho maior que 100 micrômetros). As inclusões macro são prejudiciais. As microinclusões são benéficas, pois restringem o crescimento de grãos, aumentam a resistência ao escoamento e a dureza. As microinclusões atuam como núcleos para precipitação de carbonetos e nitretos. As macroinclusões devem ser removidas. Microinclusões podem ser usadas para melhorar o fortalecimento dispersando-as uniformemente na matriz.
Os efeitos nocivos das inclusões são altamente dependentes de suas composições químicas, frações de volume, dispersões e morfologias. Inclusões normalmente grandes e inquebráveis com altos pontos de fusão são as mais indesejadas. No entanto, em comparação com essas inclusões, as pequenas e quebráveis ou aquelas com pontos de fusão mais baixos são mais preferidas. A razão para estas preferências é que as inclusões que têm pontos de fusão mais baixos ou são quebráveis são susceptíveis de serem deformadas, esmagadas em inclusões menores, ou desaparecem após processos de conformação a quente ou a frio (efeito do processo de conformação e taxa de redução) ou tratamentos térmicos que o aços sofrem após o processo de fundição e solidificação.
O comportamento mecânico do aço é controlado em grande parte pela fração volumétrica, tamanho, distribuição, composição e morfologia das inclusões e precipitados, que atuam como geradores de tensão. A distribuição do tamanho das inclusões é particularmente importante, uma vez que macroinclusões grandes são as mais prejudiciais às propriedades mecânicas. Às vezes, um defeito catastrófico é causado por apenas uma única grande inclusão em todo o calor do aço. Embora as grandes inclusões sejam em muito menos numerosas que as pequenas, sua fração de volume total pode ser maior.
Aços limpos são aqueles aços que contêm inclusões limitadas em termos de tamanho, forma, composição, distribuição e frequência. Como resultado, os aços limpos são capazes de superar outros materiais e se destacar em estados de alta tensão aplicados, como aqueles usados em equipamentos de transporte e outras aplicações.
A limpeza do aço é um fator importante da qualidade do aço e a demanda por aços mais limpos aumenta a cada ano. No entanto, o termo “aço limpo” é usado com cautela pelos metalúrgicos. Isso se deve (i) às diferentes demandas de limpeza para aços para diferentes aplicações, (ii) à variação de limpeza em aços produzidos em diferentes operações e (iii) ao entendimento normal do termo 'aço limpo', que alguns interpretam literalmente como significando a ausência de inclusões no aço. A limpeza do aço tem implicações tanto do ponto de vista operacional quanto do desempenho do produto.
As demandas cada vez maiores por alta qualidade fizeram com que os produtores de aço prestassem muita atenção aos requisitos de “limpeza” dos produtos de aço produzidos por eles. Diferentes tipos de aço estão sendo produzidos pelo produtor de aço para atender a vários requisitos esperados dos produtos de aço. O nível de limpeza do aço para cada requisito depende do número de inclusões, morfologia, composição e distribuição de tamanho de cada grau de aço. Por exemplo, em usinagem livre ou aço resulfurizado, a ideia não é remover completamente as inclusões, mas modificá-las para melhorar a usinabilidade. Portanto, uma opinião equilibrada sobre o nível permitido de inclusões ou limpeza para cada tipo de aço é realmente de grande importância técnica e econômica tanto para o produtor de aço quanto para o usuário de aço. Em grande parte, o termo “aço limpo” deve ser enfatizado por atender às especificações e requisitos do cliente para uma aplicação em relação às características de inclusão não metálica.
Com os requisitos de limpeza mais rigorosos e o desenvolvimento de novas classes de aço, é importante entender o processo de formação e evolução das inclusões e desenvolver métodos para melhorar sua remoção do aço líquido. A remoção de inclusão é favorecida não apenas por um grande tamanho de inclusão, mas também por uma alta energia interfacial entre a inclusão e o aço e grandes ângulos de contato entre a inclusão e o aço em um sistema aço-inclusão-gás.
Os requisitos de inclusão de aço limpo variam dependendo do grau e da aplicação do aço e o objetivo da engenharia de inclusão é reduzir as inclusões que são prejudiciais e promover a formação daquelas inclusões que têm efeitos benéficos.
Os avanços na fabricação de aço durante as últimas décadas resultaram em tipos de aço com níveis muito baixos de impurezas. Nos últimos anos, novos aços 'limpos' e 'ultralimpos' foram desenvolvidos e comercializados por produtores de aço em todo o mundo, respondendo assim às demandas de mercado atuais e futuras de aço com propriedades mecânicas consideravelmente melhoradas (por exemplo, resistência à fadiga e resistência ao impacto ) e uma resistência à corrosão melhorada. Esses aços podem ter um teor extremamente baixo de oxigênio (menos de 10 ppm) e enxofre (menos de 10 ppm). A força motriz por trás desses avanços tem sido o desenvolvimento de novos aços que podem tolerar aplicações altamente exigentes, por exemplo. componentes de transmissão para a indústria automotiva e peças de construção e tubos para ambientes agressivos e corrosivos.
Embora os aços de alta limpeza de hoje tenham excelentes propriedades mecânicas e/ou resistência à corrosão, esses avanços nas propriedades funcionais ocorreram às custas de quebra de cavacos mais difícil e, em alguns casos, uma vida útil da ferramenta consideravelmente reduzida em operações de usinagem.
A usinagem de aços com alta limpeza está, em geral, associada ao alto consumo de energia, maior desgaste das ferramentas de corte e altos custos de produção. Estima-se que mais de 40% do custo total de produção para produzir um componente automotivo vem de diferentes operações de usinagem. Portanto, a questão principal é avaliada como otimizar os graus de aço atuais em relação aos requisitos combinados de usinabilidade e desempenho. Portanto, as inclusões são, até certo ponto, necessárias para um desempenho de usinabilidade adequado. No entanto, o conteúdo e as características das inclusões ainda são para garantir que as propriedades de alto desempenho do aço possam ser obtidas.
Características importantes do processo siderúrgico secundário são o processo de produção, o aço líquido, os refratários da panela, os aditivos, a escória, a temperatura, o tempo e o método de tratamento. Estes são fatores cruciais que têm efeitos sobre várias características das inclusões, como mostrado na Fig 1.
Fig 1 Fatores que afetam várias características de inclusões durante a fabricação de aço
Existem três etapas no processo de formação de inclusões. Esses estágios são (i) nucleação, (ii) crescimento e (iii) coalescência e aglomeração. Na fase de nucleação, os núcleos da nova fase são formados como resultado da supersaturação da solução (aço líquido ou sólido) com os solutos (por exemplo, alumínio e oxigênio) devido à dissolução dos aditivos (agentes desoxidantes ou dessulfurantes) ou resfriamento do aço. O processo de nucleação é determinado pela tensão superficial no aço líquido de inclusão de contorno. Quanto menor a tensão superficial, menor a supersaturação é necessária para a formação dos novos núcleos de fase. O processo de nucleação é muito mais fácil na presença de outra fase (outras inclusões) no aço líquido. Neste caso, a nova formação de fase é determinada pelo ângulo de umedecimento entre um núcleo e a inclusão do substrato. A condição de molhagem (baixo ângulo de molhagem) é favorável para a nucleação da nova fase.
Na fase de crescimento, ocorre o crescimento dos núcleos. O crescimento de uma nova inclusão continua até que o equilíbrio químico seja alcançado (sem supersaturação). O crescimento de inclusões em aço sólido é um processo muito lento, portanto, um certo nível de supersaturação fora do equilíbrio pode ser retido.
A coalescência e a aglomeração ocorrem devido ao movimento do líquido devido à convecção térmica ou à agitação forçada que causa colisões das inclusões, o que pode resultar em sua coalescência (fusão de inclusões líquidas) ou aglomeração (fusão de inclusões sólidas). O processo de coalescência/aglomeração é impulsionado pela vantagem energética obtida devido à diminuição da superfície limite entre a inclusão e o aço líquido. Inclusões com energia de superfície mais alta têm maior chance de se fundir quando colidem.
A remoção da inclusão do aço líquido envolve sua flotação até a interface aço-escória, separação do aço e posterior absorção na escória. O mecanismo fundamental da flotação de inclusão no aço é pela lei de flotação de Stokes. Usando esta equação e para uma inclusão esférica de alumina de 20 micrômetros, o tempo estimado para flutuar uma distância de 2 metros é de cerca de 120 minutos. Este tempo de flotação é reduzido à medida que o tamanho da inclusão aumenta e é ainda melhorado pela agitação do argônio e a subsequente fixação das inclusões às bolhas de gás argônio. Como exemplo, inclusões de alumina com tamanho de 100 micrômetros flutuam em 5 minutos. A agitação de argônio também promove o crescimento da inclusão por colisão e subsequente aglomeração/coalescência
Grandes inclusões flutuam mais rápido do que as menores. Inclusões grandes são normalmente flutuantes e, como resultado, elas flutuam facilmente do aço para a fase de escória. Inclusões menores que não são tão flutuantes levam mais tempo para flutuar do aço. As inclusões flutuantes são absorvidas pela escória. O processo de flutuação pode ser intensificado por agitação moderada. A agitação vigorosa resulta na quebra das inclusões maiores em inclusões de tamanho menor. Bolhas de gás subindo pelo aço líquido também promovem a flutuação das inclusões e absorção pela escória.
Classificação de inclusões
As inclusões são produzidas em aço líquido durante o refino em altas temperaturas e ou por precipitação durante a solidificação. As inclusões que são produzidas durante o refino do aço em altas temperaturas são conhecidas como inclusões primárias e as inclusões que são produzidas durante a solidificação são conhecidas como inclusões secundárias. Uma vez que as inclusões são formadas no aço, as características das inclusões como tamanho, quantidade, composição e morfologia permanecem as mesmas ou mudam/evoluem devido a reações físico-químicas no aço líquido, entre o aço líquido e a escória circundante e o refratário da panela , e de deformação. Dependendo de suas características finais, podem ser prejudiciais ao processo de fundição, reduzir as propriedades mecânicas do aço e diminuir a qualidade superficial e geral do produto siderúrgico. As inclusões, cuja presença define a pureza do aço, são classificadas por conteúdo químico e mineralógico, por estabilidade e por origem.
De acordo com uma classificação tradicional, as inclusões podem ser distinguidas em duas classes principais em função de sua origem. Essas classes são (i) inclusões endógenas e (ii) inclusões exógenas. As inclusões endógenas se formam por precipitação dentro da fase líquida devido à diminuição da solubilidade das espécies químicas contidas nos aços. Esta classe de inclusões não metálicas não pode ser completamente eliminada do aço, mas a diminuição da sua fração volumétrica e do tamanho médio deve ser estrito controle para evitar a ativação dos fenômenos danosos.
Pelo contrário, as inclusões exógenas são consequência do aprisionamento de materiais não metálicos provenientes de escórias, fragmentos refratários ou de pós de subida e cobertura utilizados para proteger o aço e evitar a aderência durante a fundição do aço. As inclusões pertencentes a esta classe podem ser caracterizadas por grandes dimensões e sua origem não pode ser imediatamente reconhecível, embora sua presença possa comprometer fortemente a solidez microestrutural dos aços e a confiabilidade mecânica associada. Como as inclusões exógenas estão sempre relacionadas ao processo, elas podem ser eliminadas implementando procedimentos de processamento adequados.
Inclusões endógenas – Inclusões endógenas (também conhecidas como inclusões indígenas) ocorrem dentro do aço líquido, precipitando durante o resfriamento e a solidificação. As inclusões pertencentes a esta classe resultam de aditivos ao aço. São produtos de desoxidação ou inclusões precipitadas durante o resfriamento e solidificação do aço. Inclusões de alumina (Al2O3) em aço LCAK (alumínio de baixo carbono morto) e inclusões de sílica (SiO2) em aço morto com silício são geradas pela reação entre o oxigênio dissolvido e o alumínio adicionado e os desoxidantes de silício são inclusões de desoxidação típicas.
As inclusões de alumina são dendríticas quando formadas em um ambiente com alto teor de oxigênio (Fig. 2). As inclusões de alumina do tipo cluster por desoxidação ou reoxidação (Fig. 2) são típicas de aços mortos com alumínio. As inclusões de alumina formam facilmente aglomerados tridimensionais por meio de colisão e agregação devido à sua alta energia interfacial. As inclusões individuais no aglomerado podem ter de 1 micrômetro a 5 micrômetros de diâmetro. Antes da colisão, quebra ou agregação com outras partículas, elas podem ter a forma de placas de flores ou inclusões poliédricas (agregadas). Alternativamente, acredita-se que inclusões de alumina semelhantes a corais resultem do “maturamento de Ostwald” de inclusões de alumina originalmente dendríticas ou agrupadas. As inclusões de sílica são normalmente esféricas devido ao estado líquido ou vítreo no aço líquido. A sílica também pode se aglomerar em aglomerados.
Fig 2 Tipos de inclusões
As inclusões precipitadas se formam durante o resfriamento e a solidificação do aço. Durante o resfriamento, a concentração de oxigênio/nitrogênio/enxofre dissolvido no líquido aumenta enquanto a solubilidade desses elementos diminui. Assim, inclusões como alumina, sílica, nitreto de alumínio e sulfeto precipitam. Os sulfetos se formam interdendricamente durante a solidificação e frequentemente nucleam em óxidos já presentes no aço líquido. Essas inclusões são normalmente pequenas (menos de 10 micrômetros).
As inclusões endógenas são tipicamente distribuídas de forma mais uniforme do que as inclusões exógenas, que são aprisionamentos de materiais de interfaces refratárias, escória ou outros materiais em contato com o aço líquido. As inclusões endógenas ocorrem naturalmente e, portanto, só podem ser minimizadas e não podem ser completamente eliminadas. Óxidos primários e endógenos, como alumina e magnésio-espinela, entopem os bicos de entrada submersos e suas formas irregulares atuam como elevadores de tensão durante a deformação e diminuem a resistência mecânica do aço.
Inclusões exógenas – As inclusões exógenas surgem da interação química e mecânica não intencional do aço líquido com seu entorno. Eles são normalmente prejudiciais às propriedades mecânicas sensíveis à fratura devido ao seu grande tamanho e localização perto da superfície. A maioria dessas inclusões é formada por reoxidação na qual o aço líquido, tendo desoxidantes 'livres' (alumínio, silício, manganês ou cálcio) dissolvidos no aço líquido, capta oxigênio do contato com o ar durante o vazamento e transporte através da comporta sistema. Além disso, inclusões podem ser formadas pela reação do aço líquido com gases ou vaporização da água dos vasos de transferência pré-aquecidos inadequadamente. As inclusões exógenas são normalmente aprisionadas acidentalmente durante o vazamento, vazamento e solidificação, resultando em uma distribuição aleatória em todos os produtos de aço fundido. Essas inclusões atuam como sítios de nucleação heterogêneos para precipitação de novas inclusões durante seu movimento no aço líquido.
As inclusões exógenas surgem principalmente da interação química acidental (reoxidação) e mecânica do aço líquido com seu entorno (arrastamento de escória e erosão do revestimento refratário). Na usinagem, produzem trepidação, causando pites e goivagens na superfície das seções usinadas, quebras frequentes, além de desgaste excessivo da ferramenta. As inclusões exógenas têm as seguintes características comuns.
- O tamanho grande com inclusões de erosão refratária é normalmente maior do que o de arrastamento de escória.
- A composição do composto/multifásico é causada pelos fenômenos (i) devido à reação entre o aço líquido e a sílica, FeO e MnO na escória e o revestimento refratário com as inclusões de alumina geradas podem ficar em sua superfície, (ii) à medida que as inclusões exógenas se movem, devido ao seu grande tamanho, elas podem aprisionar inclusões de desoxidação como alumina em sua superfície, (iii) inclusões exógenas atuam como núcleos heterogêneos para precipitação de novas inclusões durante seu movimento no aço líquido e (iv) inclusões de escória ou reoxidação podem reagir com os refratários de revestimento ou desalojar mais material no aço.
- Forma irregular, se não esférica devido ao arrastamento de escória ou sílica do produto de desoxidação. As inclusões exógenas esféricas são normalmente grandes (maiores que 50 micrômetros) e principalmente multifásicas, mas as inclusões esféricas de desoxidação são normalmente pequenas e monofásicas.
- Pequeno número comparado com pequenas inclusões
- Distribuição esporádica no aço e não bem dispersa como pequenas inclusões. Uma vez que normalmente são aprisionados no aço durante o fervilhamento e a solidificação, sua incidência é acidental e esporádica. Por outro lado, eles flutuam facilmente, portanto, concentram-se apenas em regiões da seção de aço que solidificam mais rapidamente ou em zonas das quais sua fuga por flotação é de alguma forma dificultada. Como resultado, eles são frequentemente encontrados perto da superfície.
- Mais prejudicial às propriedades do aço do que pequenas inclusões devido ao seu grande tamanho.
Uma questão que substitui a fonte dessas inclusões é por que inclusões tão grandes não flutuam rapidamente depois de formadas. As possíveis razões são (i) formação tardia durante a fabricação do aço, transferência ou erosão nos vasos metalúrgicos, deixando tempo insuficiente para que eles subam antes de entrar no fundido, (ii) falta de superaquecimento suficiente e (iii) fluxo de fluido durante a solidificação induz a escória do molde aprisionamento ou re-arrastamento de inclusões flutuadas antes que elas entrem totalmente na escória.
As inclusões exógenas estão sempre relacionadas à prática e seu tamanho e composição química frequentemente levam à identificação de suas fontes, sendo suas fontes principalmente reoxidação, arrastamento de escória, erosão do revestimento e reações químicas.
A forma mais comum de grandes macroinclusões de reoxidação encontradas no aço, como aglomerado de alumina, são mostradas na Fig 2. O ar é a fonte mais comum de reoxidação, que pode ocorrer de várias maneiras, como (i) aço líquido nas misturas tundish com o ar de sua superfície superior no início do vazamento devido à forte turbulência e os filmes de óxido na superfície do líquido que flui são dobrados no líquido, formando planos fracos de partículas de óxido, (ii) o ar é sugado para o aço líquido em as juntas entre a panela e o distribuidor, e entre o distribuidor e o molde, e (iii) o ar penetra no aço a partir da superfície superior do aço na panela, distribuidor e molde durante o vazamento.
Durante este tipo de reoxidação, elementos desoxidantes, como alumínio, cálcio, silício, etc. são preferencialmente oxidados e seus produtos se desenvolvem em inclusões, normalmente de uma a duas magnitudes maiores que as inclusões de desoxidação. A solução para evitar esse tipo de reoxidação é limitar a exposição do ar ao processo de lingotamento por (i) cobertura por cortina de gás inerte utilizando um manifold de anel de aço ou anel refratário poroso ao redor das conexões entre a panela e o tundish, e entre o tundish e o molde, (ii) purgar algum gás no tundish antes de vazar, e na superfície do tundish durante o vazamento, e (iii) controlar a injeção de gás na panela para evitar a formação de olhos.
Outra fonte de reoxidação é sílica, óxido de manganês e FeO nas escórias e refratários de revestimento. Por este mecanismo de reoxidação, as inclusões dentro do aço crescem à medida que se aproximam da interface da escória ou revestimento através da reação SiO2/MnO/FeO+[Al] =[Si]/[Mn]/[Fe]+Al2O3. Esta reação leva a inclusões de alumina maiores com composição variável. Esse fenômeno afeta ainda mais as inclusões exógenas de duas maneiras, a saber:(i) essa reação pode erodir e desigual a superfície do revestimento, o que altera o padrão de fluxo do fluido próximo às paredes do revestimento e pode induzir uma quebra mais acelerada do revestimento, (ii) um grande inclusão exógena de revestimento quebrado ou escória arrastada pode aprisionar pequenas inclusões, como produtos de desoxidação, e também atuar como um núcleo heterogêneo para novos precipitados. Isso complica a composição de inclusões exógenas.
Para evitar a reoxidação da escória e do revestimento refratário, é muito importante manter um baixo teor de SiO2, MnO e FeO. Tem sido relatado que tijolos com alto teor de alumina ou zircônia contendo baixos níveis de sílica livre são mais adequados.
As operações de aciaria ou transferência envolvem a mistura turbulenta de escória e metal, especialmente durante a transferência entre vasos e, portanto, produzem partículas de escória suspensas no aço. As inclusões de escória, (10 micrômetros a 300 micrômetros de tamanho, contêm grandes quantidades de CaO ou MgO, e são normalmente líquidas à temperatura do aço líquido, portanto são de forma esférica. panelas diminui o arrastamento de escória durante o período de troca de panela. Os fatores que afetam o arrastamento de escória no aço líquido durante o processo de lingotamento contínuo são (i) operações de transferência da panela para o distribuidor e do distribuidor para o molde especialmente para vazamento aberto, (ii) vórtice em a superfície superior do aço líquido com o vórtice quando o aço líquido está em baixo nível pode ser evitado de várias maneiras, como interromper o vazamento antes do início do vórtice, (iii) emulsificação e arrastamento de escória na superfície superior, especialmente sob agitação de gás acima uma taxa de fluxo de gás crítica, (iv) turbulência no menisco no molde e (v) propriedades da escória, como tensão interfacial e viscosidade da escória. Como exemplo, a escória do molde pode ser arrastada para o líquido aço fluido devido a (i) turbulência no menisco, (ii) vórtice, (iii) emulsificação induzida por bolhas que se deslocam do aço para a escória, (iv) sucção ao longo da parede do bocal devido à diferença de pressão, (v) fluxo de alta velocidade que corta a escória da superfície, e (vi) flutuação do nível.
A tensão interfacial entre o aço e o pó de fundição líquido determina a altura do menisco de aço e a facilidade de arrastamento do fluxo. Especificamente, uma tensão interfacial de 1,4 N/m para uma escória de cal-sílica-alumina em contato com ferro puro produz uma altura de menisco de cerca de 8 mm. A tensão interfacial é reduzida a um valor baixo por espécies tensoativas como o enxofre ou por uma reação de troca interfacial como a oxidação do alumínio no aço pelo óxido de ferro na escória. A baixíssima tensão interfacial associada a uma reação química pode proporcionar turbulência espontânea na interface, através do efeito Marangoni. Tal turbulência pode criar uma emulsão na interface, criando esferas indesejáveis de escória no aço.
A erosão de refratários, incluindo areia de bloco de poço, sujeira solta, tijolos refratários quebrados e partículas de revestimento cerâmico, é uma fonte muito comum de grandes inclusões exógenas que são tipicamente sólidas e relacionadas aos próprios materiais da panela e do distribuidor. Eles são normalmente grandes e de formato irregular. As inclusões exógenas podem atuar como locais para nucleação heterogênea de alumina e podem incluir a partícula central retratada, ou agregar com outras inclusões indígenas. A ocorrência de produtos de erosão refratária ou inclusões introduzidas mecanicamente podem prejudicar completamente a qualidade de aços muito limpos.
A erosão do revestimento normalmente ocorre em áreas de fluxo turbulento, especialmente quando combinada com reoxidação, altas temperaturas de vazamento e reações químicas. Os parâmetros que afetam fortemente a erosão do revestimento são dados abaixo.
- Algumas classes de aço são bastante corrosivas (como alto teor de manganês e classes que são quase mortas e têm alto teor de oxigênio solúvel) e atacam os tijolos de revestimento.
- Reações de reoxidação, como o alumínio dissolvido no aço líquido, reduzem o SiO2 no refratário do revestimento, gerando inclusões à base de FeO que são muito reativas e molham os materiais do revestimento, levando à erosão do refratário do revestimento em áreas de alta turbulência do fluido. A extensão desta reação pode ser quantificada monitorando o teor de silício do aço líquido. Esse oxigênio também pode vir do monóxido de carbono, quando o carbono no refratário reage com aglutinantes e impurezas.
- A composição e a qualidade do tijolo têm um efeito significativo na qualidade do aço. Uma siderúrgica adaptou três tipos de materiais (Al2O3 alto, Al2O3-SiC-C e MgO-C com taxa de desgaste de 1,0, 0,34, 0,16 mm/calor respectivamente) na linha de escória, onde o refratário tende a ser danificado por escória e fluxo de fundição erosivo, e o tijolo MgO-C apresenta a maior durabilidade entre os três. O óxido de manganês ataca preferencialmente as porções contendo sílica do refratário. Grãos de alumina e zircônia de altíssima pureza podem resistir ao ataque do óxido de manganês.
- A erosão refratária rápida de aços com alto teor de manganês pode ser restringida (i) usando refratários de alumina ou zircônia de alta pureza (caros) e (ii) minimizando o oxigênio matando completamente o aço com um desoxidante forte, como alumínio ou cálcio, e impedindo a absorção de ar. Os revestimentos tundish à base de sílica são piores do que os revestimentos pulverizados à base de magnésia. Refratários de alta alumina têm sido sugeridos como os mais promissores. A incorporação de óxido de cálcio no refratário do bocal pode ajudar liquefazendo as inclusões de alumina na parede, desde que a difusão do óxido de cálcio na interface seja rápida o suficiente e a erosão do bocal não seja um problema. A erosão do bocal pode ser combatida controlando a composição refratária do bocal (por exemplo, evitando impurezas de sódio, potássio e silício) ou revestindo as paredes do bocal com alumina pura, nitreto de boro ou outro material resistente. O refratário na superfície das paredes da cobertura deve ser escolhido para minimizar as reações com o aço que criam inclusões e entupimentos.
- Velocidade excessiva do aço líquido ao longo das paredes do distribuidor, como a zona de entrada. Uma almofada pode ser usada para evitar a erosão do fundo do distribuidor, bem como controlar o padrão de fluxo. Foi sugerido que velocidades de aço líquido acima de 1 m/s são perigosas em relação à erosão.
- O contato excessivo ou o tempo de enchimento e a alta temperatura pioram os problemas de erosão. Durante um longo período de retenção na panela, as inclusões maiores podem flutuar na escória da panela. However the longer the steel is in contact with the ladle lining, the more tendency is there for the ladle erosion products. Solutions are based upon developing highly stable refractories for a given steel grade, developing dense wear resistant refractory inserts for high flow areas and preventing reoxidation.
Chemical reactions produce oxides from inclusion modification when calcium treatment is improperly performed. Identifying the source is not always easy, as for example, inclusions containing calcium oxide can also originate from entrained slag.
The agglomeration of solid inclusions can occur on any surface aided by surface tension effects, including on refractory and bubble surfaces. The high contact angle of alumina in liquid steel encourages an inclusion to attach itself to refractory in order to minimize contact with steel. High temperatures of 1,530 deg C enable sintering of alumina to occur. Large contact angle and larger inclusion size favour the agglomeration of inclusions. Due to the collision and agglomeration, inclusions in steel tend to grow with increasing time and temperature. The numerical simulation of inclusion nucleation starting from deoxidant addition and growth by collision and diffusion from nano-size to micro-size is reported. The fundamentals of alumina sintering into clusters, needs further investigation, though some studies have used fractal theory to describe the cluster morphology (features).
Another classification of the inclusions can be based on their chemical composition. The inclusions can be synthetically classified as (i) sulphides, (ii) aluminates, (iii) silicates, (iv) oxides, (v) nitrides, and (vi) complex combinations of two or more of these inclusion types. The majority of the inclusions in steels are oxides and sulphides since the content of phosphorus is very small. Silicates are very detrimental to steels, especially if it has to undergo heat treatment at a later stage. Normally nitrides are present in special steels which contain an element with a high affinity to nitrogen.
Sulphides inclusions are FeS, MnS, Al2S3, CaS, MgS, Zr2S, and others. The sulphides are frequently the consequence of the calcium treatment applied in order to modify the oxide inclusions, but the little and finely dispersed CaS highly refractory inclusions can be detrimental for the casting procedure (nozzle clogging) and for the damaging effect on steel. On the contrary the MnS non-metallic inclusions (frequently modified by the combination with CaS) are exploited for improving the cutting tool workability. In this case the MnS non-metallic inclusions are intentionally formed within the metal matrix in order to make the chipping brittle) during the tool working. This role implies that the volume fraction of the inclusions has to be significant and this aspect is the reason that excludes the application of EN10247:2003 for the estimation of the cleanliness of such a class of steels.
Aluminates inclusions normally consist of calcium aluminates obtained after the calcium treatment of the liquid steel. Calcium aluminates are 12CaO.7Al2O3 (C12A7), 3CaO.Al2O3 (C3A), and CaO.Al2O3 (CA) exist in the liquid state, whereas CaO.2Al2O3 (CA2) and CaO.6Al2O3 (CA6) are solid at steelmaking temperatures.
Silicates are present in steel like a glass formed with pure SiO2 or SiO2 with admixture of iron, manganese, chromium, aluminium, and tungsten oxides and also crystalline silicates. Silicates are the biggest group among non-metallic inclusions. In liquid steel non-metallic inclusions are in solid or liquid condition. It depends on the melting temperature.
Oxides inclusions in liquid steel are mostly produced during steel deoxidation but can also result from reoxidation and slag or refractory entrainment in the steel. These inclusions can have, single or multiple phases and compositions, spherical or irregular shape, and are either solid or liquid in the steel depending on their melting temperature.
Oxides inclusions can nucleate homogeneously or heterogeneously. Homogeneous nucleation occurs without the presence of foreign surfaces in the steel while heterogeneous nucleation occurs on foreign surfaces. Sources of foreign surfaces in liquid steel can be entrained materials, the surrounding ladle refractory, pre-existing inclusions, and or undissolved alloys. For the formation of a stable oxide, the absolute contribution of the bulk free energy to the overall energy is to be greater than the interfacial energy and this occurs at a critical oxide size. Inclusions less than this critical size are unstable and re-dissolve into the liquid steel while those which are larger than this size grow. For the heterogeneous nucleation, the presence of an existing surface reduces the critical oxide size and hence, reduces the overall free energy needed for a stable oxide to be produced. Heterogeneous nucleation is more favoured compared to homogeneous nucleation.
Important inclusion characteristics are their size, amount, composition, and morphology. After a stable oxide is produced in the steel, the inclusions grow and can also change their composition due to reactions within the steel and between the steel and surrounding slag and ladle refractory. Oxides inclusion growth occurs by diffusion of oxygen and deoxidant to the inclusion, and by agglomeration and coalescing after collision.
Oxides inclusions are FeO, MnO, Cr2O3, SiO2, Al2O3, TiO2 and others. By mineralogical content, oxide inclusions divide into two main groups namely (i) free oxides such as FeO, MnO, Cr2O3, SiO2 (quartz), Al2O3 (corundum) and others, and (ii) spinels which are compound oxides formed by bivalent and trivalent elements. Ferrites, chromites and aluminates are in this group. The fundamental tool for the description of the chemical composition of the oxide inclusions is the ternary phase diagram (CaO-SiO2-Al2O3), since this is the main system ruling the formation of these non-metallic compounds. This class of non-metallic compounds are formed by the deoxidizing elements added to the liquid steel for removing the oxygen content.
Composition, size, and distribution of precipitated oxides are greatly influenced by the deoxidants, conditions of the liquid steel, and the solidification process. Aluminum is widely accepted as deoxidant in steelmaking process. Its addition is very convenient and it effectively reduces oxygen content in liquid steel to low levels. However, the most of the steel problems can be traced to alumina or Aluminum rich oxides. Solid alumina inclusions in the liquid steel tend to rapid clustering due to their dendritic morphology. The alumina clusters hardly float to the top of the liquid steel because of their high apparent density in view of oxide clusters plus engulfed liquid steel. They are detrimental to the castability and quality of continuously cast steel.
The onset of clogging during the casting process starts when an alumina inclusion attaches to the nozzle wall. Certain types of refractories, especially the graphite-stabilized magnesia refractories, have been reported to promote agglomeration of alumina inclusions. The high contact angle between the alumina inclusions and the steel further promotes the tendency of the inclusions to agglomerate on refractories. In addition, the presence of significant amounts of alumina and MnS inclusions negatively impacts the performance of steel products. In general, oxide inclusions can cause lamellar tearing and degrade the toughness, bendability and ductility of steels.
When aluminum is added to liquid steel for deoxidation, the aluminum reacts with the oxygen to form dendritic alumina inclusions (alumina galaxy). Depending on size, the alumina inclusions formed as a result of deoxidation can be divided into macro-inclusions and micro-inclusions. Partial and complete substitution of titanium, zirconium, and / or rare earth metals for aluminum is increasingly pursued. This is done to improve the castability and the quality of the continuously cast steel through generation of finely dispersed oxides which effectively serve as heterogeneous nucleation sites for transformation and precipitation. Hence, control of the amount, size, composition and distribution of inclusions in steel is of importance.
Nitrides inclusions are ZrN, TiN, AlN, CeN and others which can be found in alloyed steel and has strong nitride generative elements in its content. The nitride generative elements are titanium, aluminum, vanadium, cerium and others. The nitride inclusions are normally formed by titanium nitride (TiN) and perform a detrimental effect worsened by the peculiar edged shape which increases the amplification of the stresses which are developed at the interface between the inclusion and the metal matrix. When TiN is present in large numbers, homogeneously distributed, and in relatively small sizes, they promote the formation of equi-axed grains which improve the mechanical strength of the cast steel. Also, the presence of a specific CaO∙Al2O3∙2SiO2 oxide (Anorthite) in stainless steel 316L has been found to improve the machining tool life. These inclusions when present, act as a lubricant by coating the machining tool tip. They also promote the breaking of machining chips.
Examples of complex combinations of two or more of these inclusion types are FeO·Fe2O3, FeO·Al2O3, FeO·Cr2O3, MgO·Al2O3, 2FeO·SiO2, FeS·FeO, MnS·MnO, Nb(C, N), V(C, N) and others.
Three main mechanisms have been recognized at the origin of the inclusions which are related to the damaging effects played by these non-metallic phases against the metal matrix. These mechanisms consider the inclusions as (i) notching elements which amplify the stress field around the inclusions, (ii) pressurized tanks of gas which progressively migrates into the inclusions generating a stress field around the inclusions, (iii) non-metallic phases which generate a residual stress due to the different thermal expansion coefficient associated to the metal phase and the glassy-ceramic ones.
The first mechanism is associated to a ductile process of crack formation which develops starting from the interface between the inclusions and the steel. The voids are the precursor of cracks and on a macroscopic level the cooperative detrimental effect related to the voids formed by a large number of inclusions produces a decrease of the ultimate tensile strain value. This relation points out that the factors detrimentally influencing the toughness and the macroscopic ductility of the steels are (i) the increase of the volume fraction, (ii) the decrease of the curvature radius, and (iii) the fracture of the non-metallic inclusions.
The coalescence among the nucleated voids is very dangerous since the voids of adjacent inclusions can coalesce to form a large crack, so the formation of elongated strips of inclusions represents an extreme situation. Hence, the inclusions constituted by the brittle ceramic phases which can form elongated fractured strips have to be carefully avoided. It is worth noting that the just described mechanism is featured by a ductile process on microscopic scale, but its effect on a macroscopic level turns out as a decrease of the toughness and of the ductility.
The second mechanism is related to the highest solubility shown for hydrogen by the sulphides. Hence, the inclusions become pressurized tanks pulling on the metal matrix and giving rise to a stress field which can be summed to the one formed by the external force applied during the service of the steel.
The third mechanism takes place as a consequence of the different thermal expansion coefficient featuring the steel and the glassy and / or ceramic structures characterizing the inclusions. The silicates, the aluminates, and normally all the oxides (except CaO and MgO) have a thermal expansion coefficient lower than one of the steel metal matrix, while the sulphides are featured by a contrary behaviour. The detrimental action is due to the residual stress generated on the interface between the inclusions and the metal matrix.
The higher the size of the inclusion the larger is the detrimental effect, so in order to prevent this mechanism the limitation of the size of the inclusion is a fundamental aspect while the overall volume fraction of the inclusion population does not play a significant role in this mechanism.
By stability, non-metallic inclusions are either stable or unstable. Unstable inclusions are those which dissolve in dilute acids (less than 10 % concentration). Unstable inclusions are iron and manganese sulphides and also some free oxides.
The formation and the control of the chemical composition of the inclusions involve the different steps of the production processes and the industrial systems through which they are performed. The production process has to be carefully implemented in each step in order to avoid problems related to (i) difficulties during the casting operation associated with the nozzle clogging between the tundish and the mould (continuous casting process) and between the ladle and the casting column (ingot casting), and (ii) detrimental effect on the mechanical properties of the steel.
There are four main treatment mechanisms for the removal of inclusions from the liquid steel. The first mechanism is the flotation of the inclusions. As per the Stokes law, because of the differences between densities of non-metallic inclusions and liquid steel, flotation leads to the removal of the inclusions. It is possible to calculate theoretically the rate of inclusion removal due to flotation. The second mechanism is the use of the magnetic stirring and argon gas injection. These two techniques assist the removal of non-metallic inclusions. Rate of inclusions entrapment by means of argon gas injection can be calculated.
The third mechanism is the calcium treatment. Calcium treatment is an effective way which can facilitate the removal of inclusions from the liquid steel. By adding calcium to the liquid steel (mostly in form of calcium silicide), it is possible to modify unmelted aluminum-magnesium rich inclusions (spinels) to large, isotropic, and spherical calcium aluminates and calcium sulphides with low melting points. This assists the removal of liquid inclusions. However, it can become a problem if for any reasons some of these large calcium aluminates remain or get trapped in the liquid steel.
The fourth mechanism is to optimize the properties of the top slag. Optimized properties of the top slag can enhance the inclusions removal in the ladle furnace. The three mechanisms mentioned above facilitate the inclusions movement from the middle or bottom parts of the liquid steel bring the inclusions to the ladle top. However without a proper top slag, it is highly probable that these inclusions cannot be removed efficiently. Hence, in order to ensure a very effective entrapment and absorption of non-metallic inclusions by means of top slag, it is necessary to have an optimized liquid top slag with high absorbing capacity for inclusions, proper wetting properties, and viscosity.
Inclusion engineering
Solid-phase inclusions can cluster together to clog nozzles and other flow control systems which mediate the flow of liquid steel, posing a threat to the process operations. Some inclusion chemistries reduce ductility, resistance to fatigue, or overall toughness in steels. The absence of inclusions poses issues as well because the ‘clean steels’ can be harder to machine, decreasing the lifespan of cutting tools, and require higher power consumption for machining. Understanding their nature is of critical importance in steelmaking operations, and ‘inclusion engineering’ is needed to be an operational focus during the process of steelmaking.
The term ‘inclusions engineering’ means the design of the inclusions so as to alleviate their harmful effects on the product properties. Inclusion engineering does not refer to removal of inclusions but it refers to modify them either in terms of chemical composition or shape so that harmful effects of the inclusions can be converted to improve the steel properties. Inclusion engineering also involves distribution of inclusion uniformly in the matrix, so that composite properties can be generated in the product. In some cases, deliberate attempts are made to form very fine inclusions (e.g. nitrides, and carbo-nitrides inclusions in hardening steel). Such inclusion can form by reaction between tungsten, titanium, aluminum with oxygen, nitrogen, sulphur, or carbon.
The approach for reducing the harmful effect of inclusions is to tailor the steelmaking process to avoid the presence of macro-inclusions while controlling the population, size, distribution, and morphology of the residual micro-inclusions in the steel. The application of new technology and the knowledge gained from end users on the performance of steel products are valuable information for use in the design of a clean steel strategy. The science of inclusion modification and shape control stems from the need to change the chemistry of the inclusions to enhance the performance of products in the field and ensure the castability during continuous casting. However, macro-size inclusions are required to be removed. In all other cases, depending on applications, inclusion can be modified to minimize their harmful effects.
As far as inclusion modification and shape control are concerned, the inclusions of interest are the endogenous type, particularly the inclusions which result from the process of deoxidation and sulphide-type inclusions. Oxides and sulphides are the two predominant inclusions in steel. The sources of oxides and sulphides are inherent to the steelmaking process. Oxygen is employed to react with the impurity elements (e.g. silicon, manganese) and carbon to generate chemical energy for the melting process. However, a significant amount of the oxygen ends up being dissolved in the liquid steel. The dissolved oxygen is required to be removed during the refining stage because of its harmful effect on the structural integrity of the finished product. Strong deoxidants, like aluminum and silicon, are normally used to scavenge oxygen from the steel. However, aluminum-killed steels routinely clog tundish well nozzles and submerged entry nozzles during continuous casting due to the residual alumina inclusions which remain in the steel.
The element which is to be added to modify the inclusions is to meet three requirements namely (i) it is to have high chemical affinity for the inclusion, (ii) it is to be able to modify the composition so that it becomes liquid, and (iii) it is to be able to modify the shape i.e. sharp edges and corner of inclusions to spherical.
The formation of the non-metallic phases is ruled by the thermodynamic relations. The oxide system represents the most difficult one to be studied because of the presence of different oxide species.
Moreover, the insertion of calcium aiming at the modification of the inclusions makes even more difficult the understanding of the interaction taking place in the steel bath. A good procedure for the engineering of the inclusions is aimed at developing low melting non-metallic oxides in order to avoid the nozzle clogging and at maintaining a prevalently glassy structure of the inclusions during the steel cooling and the successive heating imposed to perform the plastic deformation in order to avoid the formation of ceramic brittle phases. The need to stabilize the glassy structure makes interesting the formation of silicate system based on the presence of anorthite and pseudo-wollastonite which appears to be particularly favourable.
The prediction and the engineering of the oxide inclusions can be based on a powerful and simple thermodynamic model and can be divided into three main steps namely (i) computation of the oxygen potential associated with the slag, (ii) evaluation of the possibility of the development of the reactions to create some pure non metallic compounds, and (iii) definition of a hierarchy of the different reactions as a function of the associated oxygen potentials on the basis of the chemical composition of the steel.
Ductility is appreciably decreased by increasing amounts of either oxides or sulphides. Fracture toughness decreases when inclusions are present in higher-strength lower-ductility alloys. Similar property degradation from inclusions is observed in tests which reflect slow, rapid, or cyclic strain rates, such as creep, impact, and fatigue testing. Inclusions cause voids, which can induce cracks. Large exogenous inclusions can cause trouble in the form of inferior surface, poor polishability, reduced resistance to corrosion, and in exceptional cases, slag lines and laminations. Inclusions also lower resistance to HIC (hydrogen induced cracks). The source of most fatigue problems in steel are hard and brittle oxides, especially large alumina particles over 30 micrometers. Lowering the amount of large inclusions by lowering the oxygen content to 3 ppm to 6 ppm has extended the life of steel part such as bearing by almost 30 times in comparison with steels with 20 ppm oxygen. To avoid these problems, the size and frequency of detrimental inclusions are to be carefully controlled. Especially there is to be no inclusions in the casting above a critical size.
Although the solidification morphology of inclusions is important in steel castings, the morphology of inclusions in wrought products is largely controlled by their mechanical behaviour during steel processing, i.e., whether they are ‘hard’ or ‘soft’ relative to the steel matrix. The behaviour of different types of inclusions during deformation is schematically illustrated in Fig 3. ‘Stringer’ formation, type (b) and (c), increases the directionality of mechanical properties, adversely affecting the toughness and ductility in particular. The worst inclusions for toughness and ductility, particularly in through thickness direction properties of flat-rolled product, are those deforming with the matrix, like (d) in Fig 3.

Fig 3 Behaviour of inclusions during deformation
There is a lot of information available on the effect of inclusions on product performance and on the kinetic and thermodynamic phenomena associated with inclusion evolution and formation. With a careful analysis of the available information, it is possible to develop a good practice at each stage of the steelmaking process for clean steel production. However, it is not possible or even necessary to eliminate all inclusions, as certain inclusions which are detrimental to steels for one application can be entirely harmless when present for another application. Hence, steels are expected to have varying degrees of cleanliness depending on their application.
A classification for what has to be considered a macro-inclusion has not been defined in any standard. On the other hand this information can be extremely difficult to be provided, since for a round shape inclusion a diameter of 14 micrometers to 20 micrometers can be dangerous, but for edged inclusions (i.e. TiN) the dangerous size can be stated even at a lower level (2 micrometers to 4 micrometers) as a consequence of the higher stress amplification associated to the edged shape. The treatment of this aspect is further complicated by the fact that the danger level can be strongly affected by the configuration of the non-metallic system which is ruled by the chemical composition of the participating phases. Actually, a correct engineering of the inclusions can permit to realize a sulphide crown precipitated on an oxide core and this system configuration mutually compensates the expansion coefficient of the non- metallic phases, approximating the one of the steel metal matrix.
Calcium treatment – The process of reducing the harmful effect of micro-inclusions by controlling their size, shape, and properties is known as inclusion modification. A common approach to modifying oxide and sulphide inclusions to prevent clogging and minimize any negative effects on the structural integrity of steel is through calcium injection during secondary refining of the steel. Fig 4 gives schematic of inclusion modification with calcium treatment of steel.
Fig 4 Schematic of inclusion modification with calcium treatment of steel
Calcium has a strong affinity for oxygen and can therefore be used as deoxidizers. However, the use of calcium as deoxidizer is challenged by its low boiling point of 1,439 deg C, limited solubility of 0.032 % of calcium in steel at 1,600 deg C, and a high vapour pressure of 1.81 atmospheres at 1,600 deg C. These properties make it difficult and non-economical to use calcium as deoxidizers. However, combinations of calcium and aluminum or manganese / silicon deoxidation form modified primary inclusions with lower activity and melting temperatures. For this reason, in steelmaking, calcium is added to steel more as an inclusion modifier rather than deoxidizer. Most steel grades are treated with calcium using either Ca-Si alloy or Ca-Fe(Ni) mixture depending on the alloy specification. Normally this treatment is effectively done after trim additions and argon rinsing.
The extent of inclusion modification in steel is an essential feature in secondary steel refining by calcium treatment. Portion of the calcium added to the melt undergoes reaction and remain in the melt as dissolved calcium in form of inclusions or go to the slag as slag constituent. The rest escape the system in form of vapour. It is vital that the calcium added is consumed by the liquid steel to the maximum extent to make the calcium injection efficient and cost effective.
The general effect of calcium treatment on inclusions modifications are (i) manganese sulphides are reduced in number and size, and they are transformed to calcium-manganese sulphides with varying properties, (ii) aluminum oxides, which are normally hard, angular and frequently appears in clusters are reduced in number or completely eliminated and replaced with complex CaO-Al2O3 or CaO-Al2O3-SiO2 inclusions, (iii) silicates are eliminated and replaced by CaO-Al2O3-SiO2 inclusions, and (iv) complex globular CaO-Al2O3-SiO2 inclusions are formed, frequently surrounded by sulphide rim.
Calcium is being frequently employed to treat aluminum killed steels to avoid the formation of solid alumina. Calcium treatment effectively improves the castability and the quality of the continuously cast steel, but is limited for all steel products which need either high fatigue resistance in service or high cold formability in very thin gauges. This is because of the presence of the globular calcium-aluminum oxides. The aluminum, calcium, and calcium-aluminum oxides are normally several to tens of micrometers in diameter.
Rare earth metals like cerium, and lanthanum etc., have also been used to modify inclusions, but they are not as efficient as calcium due to the slow flotation (due to their weight) of the modified inclusions. In addition, lanthanum and cerium readily corrode the ladle refractories. When calcium treatment is efficiently performed, the following two primary objectives are achieved.
- The alumina and silica inclusions are converted to liquid calcium aluminate and calcium silicate, which are globular in shape because of a surface tension effect. This change in inclusion composition and shape is known as inclusion morphology control.
- The calcium aluminate inclusions retained in liquid steel suppress the formation of MnS stringers during solidification of steel. This change in the composition and mode of the precipitation of sulphide inclusions during solidification of steel is known as sulphide morphology or sulphide shape control.
The conversion of inclusions to a globular shape plays a significant role on the separation rate of inclusions. For example, it has been observed that the alumina inclusions are non-wetting in liquid steel and tend to have a higher separation rate compared to CaO-SiO2-Al2O3. This implies that, by modifying the alumina inclusions with calcium, their ability to cluster is impeded as the liquid globular inclusions formed, and as a result are wetted by liquid steel. However, the high vapour pressure of calcium with the associated intense bath stirring promotes collision and coalescence of the alumina inclusions in the liquid steel. With the aid of calcium vapour and the resulting coalescence of the alumina inclusions through collision, their removal from the steel is enhanced compared to the small non-buoyant alumina inclusions which are to first cluster on their own (without forced convection) before they are able to separate from the liquid steel. This is why unmodified small alumina inclusions separate from the liquid steel and get attached to the refractory in the tundish only well after refining is complete in the ladle.
After effective calcium treatment all oxide inclusions normally contain some amount of calcium. Effective modification of oxide inclusions in steel depends on the dissolved aluminium and oxygen content of the steel before calcium treatment. For an essential inclusion modification, a calcium lower limit of 15 ppm to 20 ppm is needed. With a CaO-Al2O3 ratio of 12:7, low melting points of 1,455 deg C of calcium aluminate inclusions are formed. These inclusions exist in the liquid state at steelmaking temperatures.
Agglomeration of alumina, calcium aluminate and CaS inclusions on tundish nozzle refractories during continuous casting can result in a premature termination of casting due to a completely clogged nozzle. Depending on the population of the inclusions in the steel, complete clogging of the nozzle can occur within minutes of the start of casting. Analysis of clogged material in the tundish nozzle typically shows the presence of solid calcium aluminate inclusions with composition rich in either Al2O3 or CaO. For avoiding clogging during continuous casting, it is important to ensure low oxygen potential is achieved during refining prior to calcium treatment. The castability of steel has been shown to be directly related to its oxygen content.
When the calcium treatment is effective, alumina inclusions are converted to molten calcium aluminates which are globular in shape. The calcium aluminate inclusions retained in the steel suppress the formation of harmful MnS inclusions during the solidification of steel by modifying MnS inclusions to spherical CaS inclusions. When alumina is modified to calcium aluminate, the reaction sequence with additional calcium additions is Al2O3 to CA6 to CA2 to CA to C12A7. The presence of liquid calcium aluminates, CA2, CA, C12A7 at steelmaking temperatures (around 1,600 deg C) results in inclusions which are much easier to float than the solid alumina inclusions and also reduce the tendency of blocking ladle and casting nozzles.
The practice is to introduce calcium-bearing agents (CaSi, CaFe, CaAl, CaC, etc.) into the steel at the end of the steel refining in the form of powder or wire injection through hollow metallic tubes. Irrespective of the calcium bearing agent employed, the quantity of calcium required for treatment in a given weight of steel depends on the alumina content, and the oxygen and sulphur levels of the steel. A sufficient amount of calcium is required to be added to react with the alumina inclusions to form calcium aluminate compounds which are liquid at steelmaking temperatures. For completely modified inclusions, the equilibrium reactions are (i) [Ca] + [O] =(CaO), (ii) [Ca] + [S] =(CaS), (iii) 7(Al2O3) + 12[Ca] + 12[O] =12CaO·7Al2O3, and (iv) [MnS] + 2[O] + CaSi =(CaS) + (SiO2) + [Mn].
The reaction in equation (iv) for the precipitation of MnS in the bulk of the liquid steel is possible in steel containing a high sulphur level. Fig 5 shows the binary phase diagram of CaO-Al2O3. The highlighted region in the figure shows the desirable composition of the calcium aluminate inclusions. Outside the highlighted region, the phases are solid at steelmaking temperatures. These phases can be the prominent constituents when there is an over- injection or under-injection of calcium. While MnS inclusions are undesirable in the steel, the formation of solid CaS inclusions is equally undesirable. In terms of clogging, solid calcium aluminate or pure CaS inclusions are just as detrimental as the alumina inclusions. They also sinter and agglomerate on nozzle refractories.
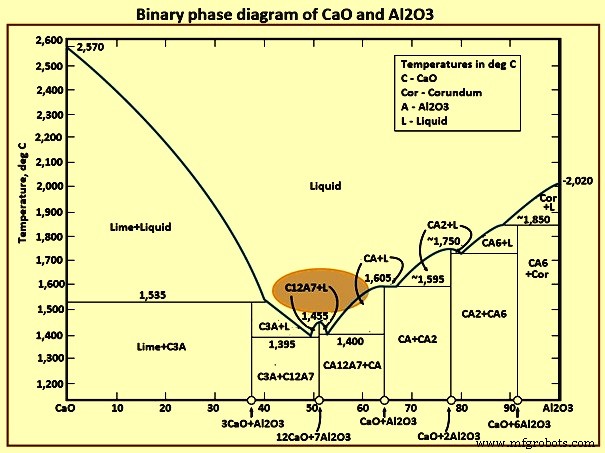
Fig 5 Binary phase diagram of CaO and Al2O3
The efficiency of calcium treatment is dependent on a number of factors, including the type, the amount and the injection rate of the calcium-bearing agent used for the treatment. Overall, by classifying the alumina and MnS inclusions according to their compositions and shapes, the efficiency of calcium treatment can be evaluated as given below.
- Class A inclusions are present when high levels of calcium have been added to the liquid steel and are liquid throughout processing. The intermingled sulphide and aluminate phases of these inclusions indicate that both phases solidified at about the same time. The sulphide phase tends to be a CaS composition. The calcium aluminate phase is either CaO·Al2O3 or 12CaO·7Al2O3. This indicates the presence of calcium aluminates with the lowest melting points and with high levels of calcium.
- Class B inclusions are the ‘bulls-eye’ type most prevalent in calcium-treated steels. The central, dark aluminate phase has solidified first, and then the outer sulphide phase precipitated onto it. In this instance, the sulphide phase tends to be (Ca, Mn)S. The calcium aluminate is of the CaO·Al2O3 or CaO·2Al2O3 composition.
- Class C inclusions are indicative of incomplete calcium treatment. These inclusions have an unmodified MnS phase, which is deformable during hot rolling. The central, dark calcium aluminate tends to be of the CaO·6Al2O3 composition, which has the lowest calcium content and remains undeformed during hot rolling.
- Class D inclusions are alumina-like oxide inclusion clusters which can have some calcium associated with them. However, there is not enough calcium present to result in complete fluxing of the alumina galaxy.
- Class E inclusions are MnS inclusions which are present when sulphur has not been completely tied up by calcium.
- Class F inclusions are inter-dendritic MnS inclusions which are present when sulphur is not completely tied up by calcium and the oxygen potential of the steel is high.
The end results of an optimized calcium treatment are:(a) the alumina is modified to form liquid calcium aluminate, and sulphur is tied up as CaS, which precipitates on the calcium aluminate inclusions, and (b) flotation of the inclusions is improved through the formation and agglomeration of spherical oxide and sulphide inclusions.
Several studies have attempted to determine the required amount of calcium addition for optimal cleanliness. For example, Ca/S ratios have been correlated to reduction of area in the Z direction and impact properties of steel. This approach cannot be generalized to all levels of sulphur. The acceptable level of Ca/S ratio in steels containing low sulphur levels can be several times higher than in steels containing higher sulphur levels, although the absolute amounts of calcium additions in the low-sulphur-containing steels are less than those of the steels containing higher sulphur levels. A good refining practice in the ladle and an efficient calcium treatment results in the majority of the alumina inclusions being converted to liquid calcium aluminate while most of the sulphur is tied up as CaS. The CaS precipitates on the calcium aluminate to produce the desirable bulls-eye shape.
Improvements of steel properties have been reported for calcium treated steel. These include (i) improvement of mechanical properties especially in transversal and through thickness direction by modifying MnS to undeformed globular (Ca-Mn)S or CaS, (ii) improvement of steel machinability at high cutting by forming protective film on the tool surface that prolongs the life of the carbide tool, (iii) improvement of surface quality and polishability, (iv) minimizing lamellar tearing in large restrained welded structures and the susceptibility of steel to reheat cracking as in the heat affected zones (HAZ) of welds, and (v) improvement of steel castability by preventing or minimizing nozzle clogging.
Processo de manufatura
- Comparando o aço das séries 300, 400 e 500
- Inconel vs aço inoxidável
- Efeito das inclusões nas propriedades do aço
- Inclusões em aço fundido contínuo e sua detecção
- Características e funções do aço ferramenta resistente ao choque
- Diferentes propriedades e classes de aço ferramenta
- Uma visão geral sobre o aço ferramenta e suas aplicações
- Entendendo o aço ferramenta e como ele é feito
- Uma visão geral do aço ferramenta e suas aplicações que o acompanham
- Uma lista de metais e ligas cobertos pelos serviços de usinagem da Gunna Engineering