Avanços e desafios dos nanomateriais fluorescentes para aplicações biomédicas e de síntese
Resumo
Com o rápido desenvolvimento da nanotecnologia, novos tipos de nanomateriais fluorescentes (FNMs) surgiram nas últimas duas décadas. A escala nanométrica confere aos FNMs propriedades ópticas únicas que desempenham um papel crítico em suas aplicações em detecções dependentes de bioimagem e fluorescência. No entanto, como a baixa seletividade e também a baixa eficiência de fotoluminescência dos nanomateriais fluorescentes dificultam suas aplicações em imagem e detecção, os cientistas ainda estão em busca de sintetizar novos FNMs com melhores propriedades. Nesta revisão, uma variedade de nanopartículas fluorescentes são resumidas, incluindo pontos quânticos semicondutores, pontos de carbono, nanopartículas de carbono, nanotubos de carbono, nanomateriais à base de grafeno, nanopartículas de metal nobre, nanopartículas de sílica, fósforos e estruturas orgânicas. Destacamos os avanços recentes dos desenvolvimentos mais recentes na síntese de FNMs e suas aplicações no campo biomédico nos últimos anos. Além disso, as principais teorias, métodos e limitações da síntese e aplicações dos FNMs foram revisadas e discutidas. Além disso, os desafios na síntese e nas aplicações biomédicas também são sistematicamente resumidos. As futuras direções e perspectivas dos FNMs em aplicações clínicas também são apresentadas.
Introdução
Os corantes orgânicos convencionais têm enfrentado algumas dificuldades em sua aplicação em biomedicina devido aos seus defeitos inerentes como citotoxicidade e baixa biocompatibilidade [1]. No entanto, o surgimento de nanomateriais fluorescentes mostra grande potencial na substituição por corantes orgânicos convencionais. Os cientistas dedicaram muito tempo e esforço na pesquisa de nanomateriais fluorescentes, e as realizações relevantes em síntese e aplicações são mais do que inspiradoras.
A forma, o tamanho e a estrutura dos nanomateriais fluorescentes determinam suas propriedades físicas e químicas, que têm grande influência em seu desempenho. Conseqüentemente, a síntese controlável de nanomateriais fluorescentes se tornou um tópico de pesquisa quente. As condições experimentais ideais de síntese contribuem para o tamanho, morfologia e estabilidade mais adequados dos nanomateriais fluorescentes. Nos últimos anos, muitos esforços foram feitos para melhorar a biocompatibilidade dos nanomateriais fluorescentes, melhorando os métodos de síntese [2]. Os íons metálicos eram geralmente dopados com pontos de carbono (CDs) ou pontos quânticos (QDs) para funcionalizar a superfície de nanomateriais fluorescentes no passado. No entanto, a fluorescência ineficaz e a toxicidade subjacente representam uma ameaça às suas aplicações em bioimagem e biomarcação [3]. Considerando esses problemas, Zuo et al. relataram um sistema de entrega de genes de CDs de alta eficiência. CDs dopados com flúor foram sintetizados por processo solvotérmico, e locais de carga positiva para entrega de genes podem ser fornecidos por polietilenoimina ramificada (b-PEI) [4]. Pode-se antecipar que novos métodos de modificação de superfície serão uma área de pesquisa de hotspot no futuro.
Muitos esforços têm sido feitos para explorar o potencial dos nanomateriais fluorescentes para aplicações biomédicas que incluem bioimagem, biodetecção e alguns métodos de terapia, conforme mostrado na Fig. 1. A fluorescência confiável para aplicação depende de suas propriedades físicas e químicas [5]. Portanto, o trabalho de pesquisa para melhorar suas propriedades, como toxicidade, hidrofilicidade e biocompatibilidade, tem sido uma parte significativa da realização do uso extensivo de nanomateriais fluorescentes em áreas biomédicas. Com o aumento da taxa de algumas doenças como o câncer, há uma demanda crescente por novos diagnósticos e estratégias de terapia com maior precisão e adesão dos pacientes [6]. Atualmente, dopagem com íons metálicos ou não metálicos e modificação de superfície de nanomateriais fluorescentes ainda são as técnicas dominantes na melhoria de sua eficiência e biocompatibilidade de PL [7], e pesquisas correspondentes abrem novas visões das aplicações biomédicas de nanomateriais fluorescentes.
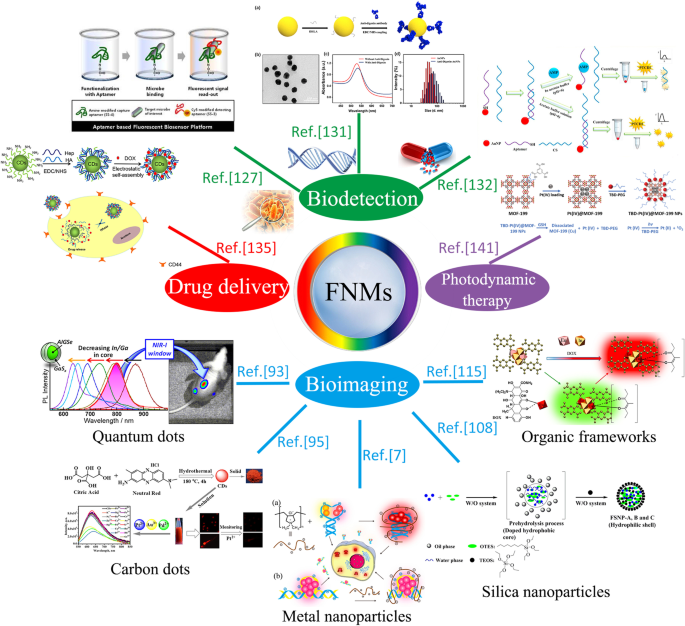
O diagrama de visão geral das aplicações biomédicas de nanomateriais fluorescentes
Considerando o grande potencial que os nanomateriais fluorescentes possuem na área biomédica, esta revisão enfatiza os avanços e melhorias mais recentes. Os cientistas têm se dedicado à funcionalização da superfície de nanomateriais fluorescentes e suas atuações em aplicações biomédicas. Somente com estratégias de síntese projetadas razoáveis os materiais fluorescentes podem ser dotados de qualidade de alta eficiência PL e boa biocompatibilidade, que são vitais para suas aplicações no campo biomédico. Esta revisão sobre a síntese e aplicações de materiais fluorescentes, esperamos, pode ser de alguma ajuda para os leitores na compreensão da tendência geral de desenvolvimento de nanomateriais fluorescentes atualmente.
Síntese de nanomateriais fluorescentes
Pontos quânticos (cristais semicondutores)
Pontos quânticos (QDs) foram o ponto de pesquisa nas últimas décadas devido à sua ampla absorção e espectros simétricos de fotoluminescência, alto rendimento quântico, alta resistência ao fotodegradação, altos coeficientes de extinção molar e grandes deslocamentos de Stokes efetivos [8]. Em termos do mecanismo de formação de QDs, quando os portadores de carga (elétrons e buracos) são restritos por barreiras potenciais a certas regiões, os semicondutores mostram efeitos de tamanho quântico dramáticos, resultando na mudança do espectro de absorção e espectro de fluorescência. As pequenas regiões são menores que o comprimento de onda de de Broglie dos portadores de carga, ou equivalentemente, o diâmetro do nanocristal é menor que o dobro do raio de Bohr dos excitons no material a granel [9]. Quando os portadores de carga são confinados por barreiras potenciais em três dimensões espaciais, QDs são formados, os quais consistem principalmente de átomos dos grupos II-VI (CdSe, ZnS), III-V (GaAs, InP), ou IV-VI (PbS, PbSe).
A síntese de QDs foi relatada pela primeira vez em 1982 [10, 11]. Nanocristais e microcristais de semicondutores foram cultivados em matrizes de vidro. Com o desenvolvimento de materiais fluorescentes, os QDs foram preparados por diferentes métodos, tais como método de adsorção direta, método de adsorção assistida por ligante, métodos situ e a combinação de métodos de preparação anteriores. A combinação de métodos anteriores inclui combinação de semicondutores preparados com precursores QD e combinação de QDs previamente preparados com precursores semicondutores, em que os semicondutores ou QDs são preparados separadamente [12].
Depois de uma série de pesquisas sobre a síntese de QDs, muitos pesquisadores relataram o estudo da propriedade fluorescente de QDs. Bawendi et al. sintetizou os QDs com distribuições de tamanho estreitas através da introdução de precursores de semicondutores, como sulfeto de cádmio (CdS), seleneto de cádmio (CdSe) ou telureto de cádmio (CdTe) para investigar as propriedades ópticas dependentes do tamanho dos QDs [13]. Desde então, CdSe se tornou a composição química mais comum de QDs, e uma variedade de modificações de superfície [14,15,16] ou invólucro inorgânico protetor [13, 17] foram utilizados para tornar a estabilidade coloidal.
Pontos de carbono
Os pontos de carbono (CDs) são nanomateriais emergentes na família dos nanocarbonos com tamanhos menores que 10 nm, que foram primeiramente obtidos na purificação de nanotubos de carbono de parede única (SWCNTs) por eletroforese em 2004 [18]. É digno de nota que os CDs substituem gradualmente os pontos quânticos semicondutores com base na alta solubilidade em água, baixa citotoxicidade, alta fotoestabilidade, emissão multicolor dependente de excitação, flexibilidade preferível na modificação de superfície, excelente permeabilidade celular e melhor biocompatibilidade [19, 20]. Geralmente, os CDs compreendem principalmente pontos quânticos de carbono (CQDs) e pontos quânticos de grafeno (GQDs). Uma grande quantidade de métodos sintéticos para CDs com tamanhos ajustáveis pode ser amplamente dividida em dois grupos principais:métodos químicos e métodos físicos [21].
Métodos Químicos Sintéticos
Os métodos de síntese química são os mais comumente usados na preparação de pontos de carbono, pois os CDs resultantes possuem excelentes propriedades, como solubilidade em água superior, inércia química, baixa toxicidade, facilidade de funcionalização e resistência ao fotobranqueamento. Em geral, os métodos de síntese química incluem síntese eletroquímica [22, 23], oxidação ácida [24, 25], carbonização hidrotérmica [26], tratamento assistido por micro-ondas / ultrassônico [27,28,29], métodos químicos de solução [30], síntese suportada [31], etc.
Entre os vários métodos sintéticos, a síntese eletroquímica foi relatada repetidamente nas últimas décadas. O grupo de Zhao relatou um novo método de preparação de CDs com baixa citotoxicidade por síntese de eletrooxidação, em que os CDs foram preparados por meio da oxidação de um eletrodo de coluna grafítica contra um eletrodo de calomelano saturado com um contraeletrodo de fio de Pt em NaH 2 PO 4 solução aquosa [22]. O sobrenadante foi então ultrafiltrado por meio de dispositivos de filtro centrífugo para a obtenção dos CDs com fluorescência azul e amarela, respectivamente. Outra abordagem eletroquímica direta foi recentemente relatada por Qu et al., Para os GQDs com um tamanho uniforme de 3-5 nm por oxidação eletroquímica de um eletrodo de grafeno em solução tampão de fosfato [23]. A cor fotoluminescente (PL) dessas partículas era verde.
Mao et al. completou a síntese de oxidação por combustão de CDs em 2007, misturando fuligem de vela com um oxidante, seguido de refluxo, centrifugação e diálise para purificar os CDs. Os espectros de fotoluminescência de CDs preparados têm uma ampla faixa de cores, com os comprimentos de onda de pico de emissão variando de 415 (violeta) a 615 nm (laranja-vermelho). Em seguida, os CDs obtidos foram posteriormente submetidos à eletroforese em gel de poliacrilamida para separar os CDs com diferentes caracterizações ópticas. A oxidação ácida também tem sido amplamente utilizada para a preparação de nanomateriais estáveis, como pontos de carbono. Após o tratamento ácido de nanotubos de carbono / grafite e refluxo, os CDs resultantes de 3-4 nm apresentaram um líquido transparente castanho-amarelado que emitia fluorescência amarela brilhante sob luz ultravioleta e eram bastante estáveis em solução salina. Isso fez CDs com fluorescência de onda longa (amarelo / laranja / vermelho) possuírem melhor penetração. A solução de CD pode ser preservada em temperatura ambiente por um longo tempo e não se formam precipitados que causem a perda da fluorescência [25].
A síntese por micro-ondas / ultrassom tem se tornado gradativamente e principalmente uma tecnologia sintética auxiliar no processo de síntese [32]. CDs fluorescentes, de 3 a 5 nm de diâmetro, foram sintetizados pelo grupo de Xiao por meio de uma abordagem econômica, rápida e verde assistida por micro-ondas [33]. A característica mais saliente dessa abordagem de uma etapa foi que a formação e a funcionalização de CDs foram concluídas simultaneamente por meio da pirólise de microondas derivada de líquidos iônicos pela primeira vez [34]. O processo de reação ocorreu em um forno de microondas usando líquidos iônicos baratos como fonte de carbono e a solução mudou de incolor para marrom escuro com o passar do tempo de reação [35]. Tang et al. usou um método ultrassônico com base em glicose ou carbono ativo como fonte de carbono para sintetizar CDs solúveis em água monodispersos. Eles emitiram fluorescência brilhante e colorida [28]. Da mesma forma, Vanesa Romero et al. obteve nitrogênio altamente fluorescente (N) e enxofre (S) pontos de carbono co-dopados (CDs) após a oxidação fotoquímica dos carboidratos em vegetais. A co-dopagem de N e S aumenta o número de sítios ativos na superfície do CD, melhorando assim seu desempenho de luminescência [36]. Pontos quânticos de carbono dopados com nitrogênio (NCQDs), uma sonda fluorescente, foram aplicados com sucesso na determinação de doxiciclina [37]. Pathak et al. preparou pontos de carbono co-dopados com nitrogênio e enxofre (NSCDs) também, que foram sintetizados a partir de tioureia e tampão tris-acetato-etilenodiamina pelo método hidrotérmico de microondas. Os NSCDs foram colocados em uso para gerar imagens de várias bactérias patogênicas e células epiteliais bucais humanas devido à fluorometria multicolorida [38].
Considerando que a maioria dos métodos sintéticos mencionados acima precisava de ácido forte, várias etapas experimentais complicadas e modificações adicionais com outros compostos para melhorar a solubilidade em água dos CDs e aumentar sua propriedade de fotoluminescência, algumas equipes de pesquisa exploraram a carbonização hidrotérmica de fotoluminescência de carboidratos, como quitosana, glicose, ácido cítrico, etc. para evitar os processos de purificação e funcionalização complexos e demorados [39]. Yang et al. descreveram um método sintético de uma etapa para CDs fluorescentes altamente funcionalizados com amino com um rendimento quântico (QY) de 7,8% por carbonização hidrotérmica de quitosana em uma temperatura amena. Este método não precisava de um solvente de ácido forte nem de um reagente de passivação de superfície. Além disso, os grupos funcionais na superfície dos CDs melhoraram sua solubilidade em água e reduziram sua biotoxicidade potencial [26]. Os pontos de carbono multi-dopados (MCDs), com emissão brilhante e com cor ajustável, foram sintetizados pelo método de um único vaso sem qualquer passivação de superfície adicional. Os MCDs sintetizados foram dopados com abundantes elementos biogênicos (O, N, P) e, portanto, apresentam forte emissão fluorescente e característica dependente do comprimento de onda de excitação, boa solubilidade aquosa, alta estabilidade óptica, bem como estabilidade iônica. Os MCDs não só podem detectar Fe 3+ de maneira seletiva e sensível sob luz azul de detecção em 15,9 nm, mas também meça a Fe intracelular 3+ por meio de imagens de fluorescência multicoloridas [40].
Para métodos de química de solução, a condensação oxidativa de grupos arila foi aplicada com sucesso à preparação de GQDs nas últimas décadas. GQDs coloidais estáveis com tamanhos e estruturas desejados foram produzidos pelo grupo de Li com estratégia de solubilização. Este método alcançou ajuste de tamanho e distribuição de tamanho estreito de CDs sem qualquer processo de separação de tamanho impraticável [30]. Quando se trata do procedimento sintético com suporte, várias equipes de pesquisa aproveitaram-se dele para concluir a síntese de nanomateriais monodispersos, como CDs nanométricos. O grupo de Zhu adotou esferas de sílica mesoporosa (MS) como nanorreatores e ácido cítrico como o precursor de carbono e CDs hidrofílicos com os tamanhos de 1,5-2,5 nm foram preparados por um método de impregnação. Os CDs com alta eficiência fotoluminescente de 23% foram capazes de emitir forte luminescência azul e apresentar excelentes propriedades de conversão luminescente [31]. Pontos de carbono emissivo amarelo brilhante (Y-CDs) foram preparados por Yan et al. pelo método solvotérmico, utilizando ácido cítrico anidro como fonte de carbono e 2,3-fenazinodiamina como fonte de nitrogênio. Os Y-CDs com abundantes grupos carboxila exibiram um rendimento quântico de fluorescência respeitável (24%), deslocamento de Stokes de 188 nm, alta sensibilidade e excelente estabilidade [41]. Os métodos sintéticos e as propriedades dos CDs são apresentados na Tabela 1.
Métodos físicos sintéticos
Em geral, os métodos físicos sintéticos incluem principalmente descarga de arco, ablação / passivação a laser e tratamento com plasma. Xu e colegas de trabalho oxidaram a fuligem de descarga de arco com HNO 3 e, em seguida, separou a suspensão por eletroforese em gel em SWCNTs. Eles finalmente isolaram a banda de movimento rápido de nanopartículas de pontos de carbono altamente fluorescentes [18]. CDs usando materiais de nano-carbono como o precursor e um solvente amigo do ambiente como o meio líquido foram preparados por Li et al. por meio de uma abordagem de ablação a laser leve [44]. Além disso, Gokus e colaboradores demonstraram que o uso de plasma de oxigênio pode induzir a fluorescência forte em grafeno de camada única [45].
Nanopartículas de carbono
Nanopartículas de carbono fluorescentes, com sua citotoxicidade reduzida, resistência ao fotobranqueamento e maior biocompatibilidade, estão atraindo cada vez mais atenção para bioimagem e outras aplicações biomédicas. Comparado com os tamanhos típicos de pontos de carbono dentro de 1-6 nm, os tamanhos das nanopartículas de carbono são mais de 20 nm, o que evita o trabalho de separar, purificar e coletar [46]. Os métodos de síntese para nanopartículas de carbono são semelhantes aos pontos de carbono, incluindo carbonização hidrotérmica, tratamento por micro-ondas, método de ablação química e ablação a laser. Esses métodos têm suas próprias vantagens, mas não podem controlar efetivamente o tamanho das nanopartículas. A carbonização eletroquímica é um método de etapa única que pode controlar o tamanho e as propriedades de luminescência das nanopartículas de carbono. Infelizmente, existem poucos substratos disponíveis para este método. No momento, alguns novos métodos intrigantes foram relatados, como o método de combustão do pentóxido de fósforo [47].
Nos últimos anos, nanopartículas de carbono adequadas para aplicações biomédicas são sintetizadas com métodos modificados. Santu et al. resolveu a síntese de nanopartículas de carbono fluorescentes vermelhas de alta qualidade por carbonização controlada de resorcinol [48]. Esta abordagem envolve o acoplamento de fenol oxidativo associado à desidratação para formar nanopartículas de carbono fluorescentes vermelhas. Anara et al. nanopartículas de carbono fluorescentes sintetizadas com rendimento quântico de 6,08% usando um método hidrotérmico modificado. Comparado com os métodos convencionais que requerem tratamento térmico longo de várias horas, este método encurtou o tempo de reação para menos de 30 min, realizando a síntese rápida de nanopartículas de carbono fluorescentes [46].
Nanotubos de carbono
Nanotubos de carbono unidimensionais (1D) têm gerado enorme atenção no campo biomédico em virtude de suas excelentes propriedades eletrônicas e ópticas. Os nanotubos de carbono podem ser divididos em nanotubos de carbono de parede única (SWCNTs) e nanotubos de carbono de paredes múltiplas (MWCNTs) de acordo com o número de camadas cilíndricas de grafeno. Enquanto os SWCNTs são compostos de uma única camada de folha de grafeno enrolada em um cilindro, os MWCNTs compreendem várias camadas concêntricas de folha de grafeno. O diâmetro externo dos nanotubos de carbono é inferior a 100 nm, mas seus comprimentos podem chegar a vários milímetros, o que leva a uma relação de aspecto muito alta e grande área de superfície [49]. Além disso, o arranjo único de átomos de carbono em nanotubos de carbono forma uma rica conjugação de elétrons π fora do nanotubo [50]. Além disso, os nanotubos de carbono são dotados de forte absorção e fluorescência na região NIR [51]. Todas essas características contribuem para uma interação efetiva com biomoléculas, o que torna os nanotubos de carbono um candidato ideal para aplicações biomédicas.
Os métodos sintéticos têm grande influência no diâmetro, comprimento, estrutura, quiralidade e qualidade dos nanotubos de carbono e, entretanto, deve-se considerar se esse método é passível de produção em larga escala. Os métodos comumente usados incluem descarga de arco [52], ablação a laser [53] e deposição química de vapor [54]. Além disso, os nanotubos de carbono precisam ser funcionalizados para melhorar sua solubilidade e evitar que se agregem em solventes e meios biológicos. A funcionalização covalente introduziria defeitos na estrutura dos nanotubos de carbono, levando à diminuição dramática ou mesmo à perda completa de sua fluorescência NIR. A funcionalização não covalente com moléculas anfifílicas, como polímeros, preservaria a estrutura e as propriedades fluorescentes dos nanotubos de carbono, mas diminuiria o QY dos nanotubos de carbono. A fim de superar esses obstáculos, novos métodos para sintetizar e funcionalizar nanotubos de carbono foram relatados recentemente. Lee et al. relataram que a adição de ditiotreitol, que é um agente redutor, pode aumentar o QY fluorescente de SWCNTs pela primeira vez, resultando em fluoróforos com brilho equivalente ao de QDs [55]. Hou et al. investigaram a adição de ditiotreitol a SWCNTs funcionalizados com uma variedade de surfactante. Para SWCNTs envolvidos em DNA e SDS, o QY fluorescente deles aumentou significativamente, enquanto a extinção da fluorescência em diferentes extensões foi observada para outro surfactante [56]. Como resultado, a adição de ditiotreitol ao DNA ou SWCNTs envoltos em SDS são soluções viáveis para alcançar a aplicação de nanotubos de carbono na biomedicina.
Nanomateriais à base de grafeno
Como nanomateriais de carbono bidimensionais, o grafeno e seus derivados têm sido amplamente explorados para uma gama de aplicações biomédicas, como bioimgaing e distribuição de drogas. Os nanomateriais de grafeno incluem nanofolha de grafeno, óxido de grafeno (GO) e nanofolha de óxido de grafeno reduzido (rGO). Eles têm grandes áreas de superfície e propriedades de superfície únicas que permitem interações não covalentes com moléculas de corante, biomoléculas e drogas insolúveis em água. Muitos pesquisadores relataram diferentes métodos de preparação de grafeno desde que foi preparado com sucesso pela primeira vez em 2004. Os métodos sintéticos de nanomateriais de grafeno podem ser classificados em duas categorias, top-down e bottom-up.
Métodos top-down envolvem o isolamento de camadas de grafite empilhadas para formar folhas de grafeno, incluindo esfoliação mecânica [57], esfoliação à base de solvente [58] e esfoliação eletroquímica [59]. Gu et al. estudaram sistematicamente a esfoliação à base de solvente assistida por ultrassom e descobriram que as ondas ultrassônicas têm um bom efeito de esfoliação. Eles também podem afetar o tamanho e a distribuição da espessura das folhas de grafeno, o que torna a síntese controlável possível. As abordagens ascendentes envolvem a reorganização dos átomos de carbono usando fontes alternativas de carbono. O crescimento epitaxial [60] e a deposição de vapor químico (CVD) [61] são os métodos de síntese ascendentes mais comumente usados. Folhas GO compostas de muitos sp 2 domínios isolados por grupos contendo oxigênio podem ser sintetizados usando o método de Hummer. Variações nos tamanhos destes sp 2 domínios fazem o PL das folhas GO variar amplamente de 500 a 800 nm [62]. O rGO é derivado do GO por redução química usando agentes redutores como hidroquinona e hidrazina. Comparado com GO, a fluorescência de rGO mostrou emissão desviada para o azul na região de UV junto com extinção fluorescente, que é atribuída às vias de percolação entre o sp cristalino recém-formado 2 clusters [63]. Akbari et al. elucidou que a proporção de sp 3 / sp 2 domínios em folhas GO determinam seus espectros de fluorescência. Portanto, GO é um nanomaterial fluorescente promissor em uma ampla faixa de comprimentos de onda sob diferentes graus de redução, que pode ser usado em aplicações biomédicas.
Nanomateriais de metal
Átomos de metais nobres apresentam menos citotoxicidade em comparação com QDs ao mesmo tempo. Nanopartículas de ouro, prata e cobre têm recebido atenção crescente e são aplicadas em um grande número de campos. Nos campos biomédicos, os efeitos da mecânica quântica das nanopartículas de ouro, como a emissão de fotoluminescência ou ressonância de plasmon, tornam as nanopartículas de ouro (AuNPs) um candidato ideal para outro nanosensor in vivo com baixa citotoxicidade [64, 65].
AuNPs têm atraído amplo interesse científico em virtude de sua facilidade de síntese e propriedades únicas, e diversos métodos sintéticos foram relatados. Como um dos métodos mais importantes, os métodos químicos são geralmente realizados tratando uma solução aquosa de cloroaurato com agentes redutores na presença de um agente estabilizador. O ácido cítrico é amplamente utilizado, o que pode atuar tanto como um estabilizador quanto como um agente redutor [66]. No entanto, AuNPs estabilizados com ácido cítrico podem sofrer acúmulo irreversível durante o desenvolvimento da funcionalização com ligantes tiolato. Este problema pode ser superado fazendo com que a reação ocorra na presença de polímeros solúveis em água, surfactantes ou agentes de cobertura que ajudam a fornecer maior estabilidade e prevenir a agregação de nanopartículas. O tamanho e a forma dos AuNPs podem ser controlados alterando a proporção de ouro-citrato, agentes modificadores de superfície ou condições de reação. Com o método de emulsificação ultrassônica em um único recipiente, Zhang e seus colegas carregaram Bis (4- (N- (2-naftil) fenilamino) fenil) -fumaronitrila e AuNPs em micelas para obter a nanossonda [67]. Acima de tudo, a nanossonda obtida, com grande potencial para ser aplicada em imagens e diagnósticos direcionados a tumores in vivo, processou excelente capacidade de imagens de fluorescência, apesar da existência de nanopartículas de ouro. Embora AuNPs não sejam tóxicos sob certas condições experimentais, a toxicidade e os efeitos colaterais precisam ser examinados minuciosamente [68].
Nanoclusters de Ag fluorescentes têm recebido muita atenção devido às suas propriedades físicas e químicas únicas. O processo de síntese de tais nanoclusters é classificado pelo arcabouço de estabilização em oligonucleotídeos de DNA, peptídeos, proteínas, dendrímeros e polímeros. Além disso, extensa literatura tem demonstrado alguma síntese verde, como a aplicação de extratos aquosos de caule de D. trifoliata e S. alba para otimizar as condições de preparação [69].
Nanoclusters de Cu (Cu NCs) são relativamente amplamente utilizados como materiais de metais nobres, mas sua síntese ainda é escassa devido à sua vulnerabilidade à oxidação. Recentemente, Kawasaki et al. preparou com sucesso Cu NCs estáveis por um método de poliol assistido por micro-ondas [70]. O DNA pode ser usado como molde para a síntese de Cu NCs fluorescentes. Mohir et al. propuseram um método baseado em DNA de fita dupla em solução para a obtenção de Cu NCs com alta seletividade [71]. Usando propriedades fluorescentes de Cu NCs, foi explorado com sucesso como um indicador de sinal fluorescente de ativação eficaz para a determinação seletiva de RDX [72].
Nanopartículas de sílica
Considerando as propriedades de transparência, estabilidade mecânica, robustez e estabilização dos fluoróforos incorporados, as nanopartículas de sílica são amplamente aplicadas em domínios biológicos. Por exemplo, NPs de núcleo / casca de sílica aplicados para a detecção intracelular de Zn 2+ e H 2 PO 4 - em células vivas foram sintetizados como nanossensores fluorescentes “desligados / ligados”. Nos últimos anos, nanopartículas de sílica dopadas com corantes orgânicos foram sintetizadas e amplamente utilizadas em muitas aplicações, como biodetecção [73]. Os métodos de síntese mais amplamente utilizados para nanopartículas de sílica são o método de Stöber e o método de microemulsão reversa. O método de Stöber, descrito pela primeira vez na década de 1960 [74], envolve a hidrólise de alquil silicatos e a subsequente condensação do ácido silícico em soluções alcoólicas catalisadas pela adição de amônia. O segundo método de formação de NP de sílica, o método de microemulsão reversa, envolve a reação de alquil silicatos, tipicamente TEOS, dentro das gotículas de água de uma microemulsão de água em óleo [75]. He et al. preparou três tipos de nanopartículas de sílica de dopagem de corante com método de Stöber e método de microemulsão reversa incorporando corante no núcleo da partícula. Algumas moléculas respondem funcionalmente ao Zn 2+ são depositados nas superfícies das partículas [76]. As nanopartículas de sílica fluorescentes foram usadas para imagens fluorescentes Zn intracelular 2+ (H 2 PO 4 - ) em células HeLa. Quando Zn 2+ foi adicionado ao Zn proporcional 2+ nanossensor, as nanopartículas mostraram a capacidade de detectar raciometricamente a concentração de H 2 PO 4 - .
Em geral, nanocompósitos de sílica com boa monodispersidade e biocompatibilidade podem ser facilmente modificados posteriormente com grupos funcionais [77,78,79]. Lee et al. dopou nanopartículas magnéticas e corantes fluorescentes em nanopartículas de sílica. Esses nanocompósitos de sílica podem ser usados não apenas como sondas de imagens multimodais para ressonância magnética (MR) e imagens de fluorescência, mas também como transportadores de liberação de drogas anticâncer [80]. Em suma, as partículas de sílica podem ser um ponto de pesquisa em casos com utilizações muito extensas.
Fósforos
Os fósforos são amplamente utilizados na biomedicina devido às suas vantagens únicas na redução da autofluorescência e da interferência de dispersão de luz dos tecidos. Em geral, os fósforos são compostos de materiais hospedeiros e íons dopados [81]. Entre os materiais hospedeiros de fósforos, óxido de ítrio (Y 2 O 3 ) é mais do que promissor devido à sua baixa fotodurabilidade e sua energia de fônon. Os lantanídeos são amplamente dopados com fósforos, considerando seus abundantes níveis de elétrons e canais de transferência de energia. Numerosos métodos foram relatados para a preparação de fósforos, incluindo hidrotérmico [82], pirólise por spray de chama [83], sol-gel [84] e processos de co-precipitação [85].
A síntese hidrotérmica surge como um processo ideal, que tem se mostrado eficiente e econômico para a síntese de fósforos. Yu et al. sintetizado Y 2 O 3 :Eu 3+ fósforos por método hidrotérmico na presença de citrato de sódio [82]. O concentrado de citrato de sódio, a quantidade de adição de NaOH e Eu no processo hidrotérmico decidiram as propriedades dos fósforos obtidos. A pirólise por spray de chama é um método promissor para a síntese rápida e consecutiva de fósforos baseados em óxidos. Comparado com os métodos convencionais, este método fornece fósforos com alta cristalinidade e distribuição homogênea de dopante. Khan et al. produzido com sucesso Tb 3+ –Dopou Y 2 O 3 fósforos que tinham aproximadamente 100 nm de diâmetro com uma distribuição de tamanho estreita usando pirólise de spray de chama [83]. Em seu método, o sal alcalino foi misturado com outros precursores de nitrato de metal, o que efetivamente controlou a distribuição de tamanho em uma faixa estreita. A rota de síntese sol-gel oferece várias vantagens, como alta homogeneidade e pureza, tempo de síntese reduzido, morfologia de partícula uniforme e distribuição estreita de tamanho de partícula [86]. Leonardo et al. obteve Sm 3+ dopado SiO 2 -Gd 2 O 3 phosphors by a sol–gel process [84]. Co-precipitation is a common and simple method for synthesizing crystalline phosphors, which ensures high homogeneity and controlled morphology characteristics. Perhaita et al. reported that the phase composition of the phosphors strongly depends on the pH during the precipitation [85].
Organic Frameworks
Covalent-organic frameworks (COFs) are new porous crystalline materials possessing outstanding stability, adsorption and low toxicity. The design of fluorescent small organic molecules with a combination of fluorescence determination methods can be used to construct more efficient nanoprobes [87]. For selective 2,4,6-trinitrophenol (TNP) determination, a novel Naphthalimide-Benzothiazole conjugate was prepared as colorimetric and fluorescent nanoprobe. The fluorescence emission peaks of receptor were selectively quenched by TNP with a limit of detection as low as 1.613 × 10 –10 M.
Metal organic frameworks (MOFs) are a kind of new generation multifunctional inorganic–organic materials with various holes and functionalized 3D crystalline structures formed by metal ions and linkers. MOFs show potential applications in separation, catalysis and other aspects due to unique attributes such as excellent chemical tenability, specific surface area and confinement of the pores. Some of the MOFs are luminescent and the quantum yield as well as light intensity will be influenced by temperature and excitation wavelength [88]. With the addition of doxycycline, Yu et al. synthesized a new functional metal–organic framework of pyromellitic acid and europium, which exhibited remarkable fluorescence enhancement at 526 nm and 617 nm. Results showed that both fluorescence intensities were positively correlated with the doxycycline concentration. The unique fluorescence response of the system could discriminate doxycycline from other tetracycline antibiotics with high selectivity.
Biomedical Applications of Fluorescent Nanomaterials
Bioimaging
Quantum Dots for Bioimaging
Fluorescent nanomaterials have been widely used in bioimaging. Compared with conventional organic fluorescent molecules, fluorescent nanomaterials are equipped with many superior properties such as high photostability, tunable emission spectra and high quantum yields [89].
As early as 1998, QDs were first successfully applied in biological imaging [90]. Since then, applications of QDs in this field have been springing up gradually. Chen’s group applied it in bioimaging and nuclear targeting with great stability and biocompatibility in living cells [91]. In spite of the extremely high sensitivity and spatial resolution of QDs, poor performances on hydrophilicity and biocompatibility hindered their applications in bioimaging in vivo. To tackle this problem, it has been found that the water-solubility of QDs can be greatly improved by attaching thiol or other hydrophilic groups to the surface of quantum dots [92]. In the same way, with the intention of improving the effectiveness and specificity of in vivo targeted imaging, targeting molecules are attached to the surface of QDs. Furthermore, the wavelength region of the emission light can be controlled by altering the size of QDs.
The combination of QDs and inorganic metal ions can optimize the application of QDs in bioimaging because the QDs’ defect-site PL peaks will be utterly removed by controlling the proportion of doped inorganic metal ions. Kuwabata, S et al. modulated the degree of Ga 3+ doping in Ag–In–Se QDs. Thus, the QDs’ defect-site PL peaks were completely removed and a sharp band-edge emission peak come into appearance [93]. They found a blue shift of the band-edge PL peak ranging from 890 to 630 nm which could be credited to the fact that the energy gap of QDs was enlarged by Ga 3+ doping. After injecting a mouse with QDs, the potential of AIGSe@GaSx core–shell QDs for bioimaging turned out satisfying. The imaging effect of this kind of QDs in mice is demonstrated in Fig. 2. However, sensing of mid-IR wavelengths is challenging due to increased dark currents and noise. HgTe QDs synthesized by colloidal method is a promising candidate for IR bioimaging by virtue of lower dark currents, higher-temperature operation, and higher detectivity [94].
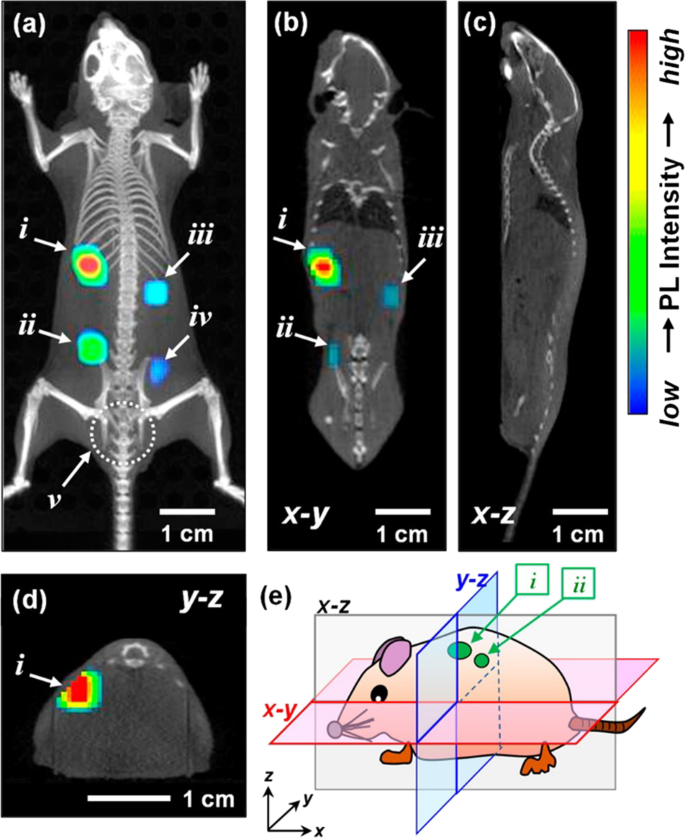
Three-dimensional PL image superimposed on an X-ray CT image of the mouse subcutaneously injected with DSPC-AIGSe@GaSx liposome dispersions (each 50 mm 3 ) in the back [78]
Carbon Dots for Bioimaging
The poor photostability of current fluorescent nanomaterials hinders their long-term bioimaging to a large extent. To overcome this limitation, CDs have been studied for bioimaging and some positive results have been obtained due to the great performance on PL efficiency. Enormous efforts have been put to improve their water solubility and lower their toxicity in organisms. At present, most CDs are facing a barrier in bioimaging, that is, their short-wavelength excitation disables deep penetration in tissue. Aside from this, being exposed under the short-wavelength for a long time could do irreversible damages to living cells and tissues. As shown in Fig. 3, with the purpose of overcoming this deficiency, Gao et al. designed fluorescent CDs with red emission which were successfully used for bioimaging of noble metal ions (Pt 2+ , Au 3+ , Pd 2+ ) in cells and zebrafish [95]. Sun and co-workers first studied the near infrared (NIR) imaging of CDs in vivo using mice as a model. Recently, it was reported that molecules or polymers containing plentiful sulfoxide or carbonyl groups can enhance NIR fluorescence through the surface modification. As shown in Fig. 4, under NIR excitation, sulfoxide or carbonyl groups are bound to the outer layers and the edges of the CDs. Thus, electron transitions are promoted, influencing the optical bandgap [96].
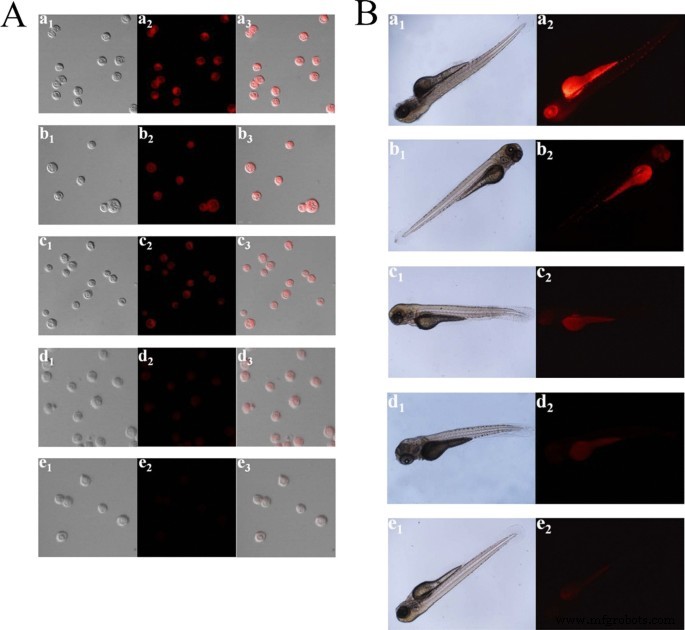
A Confocal imaging of Pt 2+ in PC12 cells. (a1–e1) Bright field images. (a2–e2) Black field images of the CDs in PC12 cells with the different concentrations of Pt 2+ (0, 25, 50, 150, and 300 μM). (a3–e3) Overlay images. B Fluorescence imaging of Pt 2+ in ZF. (a1–e1) Bright field images. (a2–e2) Fluorescence images of the CDs in ZF with the various concentrations of Pt 2+ (0, 30, 60, 100, and 150 μM) [80]
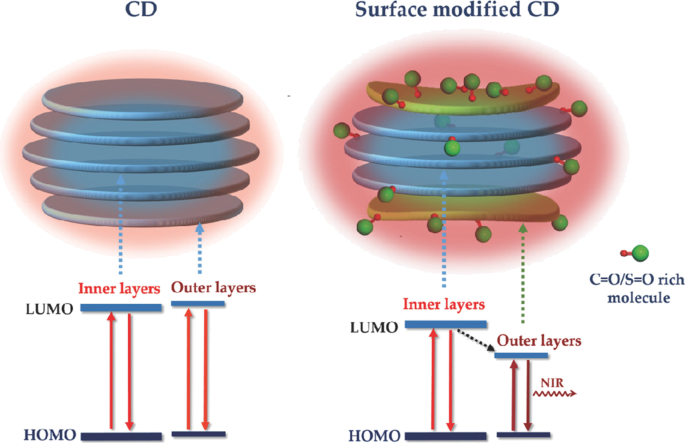
Schematic of structure and energy level alignments of nontreated CDs (left column) and CDs modified with S = O/C = O‐rich molecules (right column). The red (oxygen atom) and green double‐bonded balls represent the C = O/S = O‐rich molecule [81]
Carbon Nanoparticles for Bioimaging
In the field of bioimaging, fluorescent carbon nanoparticles show unique chemical and optical properties over traditional fluorescence probes. Different size, shape and elemental composition make carbon nanoparticles with different features. The biomedical fields are always seeking the most promising fluorescent carbon nanoparticles. Gaurav et al. obtained both larger and smaller size carbon nanoparticles with laser ablation method [97]. Both green and blue fluorescence were observed in the cells incubated with the carbon nanoparticles, suggesting their different sizes. Cell viability results indicated that the prepared carbon nanoparticles were nontoxic and safe for bioimaging applications. Shazid et al. employed carbonization method to obtain fluorescenct carbon nanoparticles derived from biocompatible hyaluronic acid. Both the in vitro and in vivo bioimaging studies showed that the prepared carbon nanoparticles would be reliable and stable for opticle imaging. Moreover, based on the experimenal data, their cytotoxicity was proved to be tolerable for biomedical applications.
Carbon Nanotubes for Bioimaging
Fluorescence of carbon nanotubes in the NIR is attracting high attention for their good light penetration depth in biological tissues. However, their low quantum yield requires for considerable excitation doses, leading to a fair degree of blue-shift and failure of penetrating live tissue. Mandal et al. reported that bright and biocompatible p-nitroaryl functionalized SWCNTs, encapsulated in phospholipid-polyethylene glycol, are suitable for bioimaging applications. The prepared SWCNTs enabled high signal-to-noise ratio imaging in live brain tissues using ultra-low excitation intensities. Their 1160 nm emissions in the NIR guarantee that they will provide optimal fluorescence imaging results [98]. Ceppi et al. applied SWCNT-based fluorescence imaging to debulking surgery in an ovarian cancer mouse model. SWCNTs are coupled to an M13 bacteriophage carrying modified peptide binding to the SPARC protein, which is overexpressed in ovarian cancer, leading to real-time imaging to guide intraoperative tumor debulking. This imaging system enables detection in the NIR window with a pixel-limited resolution of 200 μm, demonstrating real potential in fluorescence imaging guided surguries for patients [99].
Furthermore, fluorescent moieties can be conjugated by a carbon nanotube backbone, which integrate strong fluorescent ability with robust mechanism strength, exhibiting ideal bioimgaing results. Katharina et al. functionalized SWCNTs with an amphiphilic C18 -alkylated polymer conjugated with bright perylene bisimide fluorophores. The polymers wrapping around the SWCNT backbones not only increase their water dispersibility but also promote their biocompatibility by providing a shield. In vitro studies on HeLa cells demonstrated that the biocompatibility of SWCNTs is dramatically improved. In microscopy studies, direct imaging of the SWCNTs' cellular uptake via perylene bisimide and SWCNT emission proved their potential for bioimging [100]. Park et al. combined carbon nanotubes with mussel adhesive proteins which can be specifically targeted at tumors in tissue. They then made carbon nanotubes conjugated with a ZW800 NIR fluorophore to obtain NIR fluorescence imaging [101]. The prepared carbon nanotube probes react with a specific tumor in one hour and can be easily eliminated via urine, demonstrating great value as tumor imaging and detecting agent.
Graphene-Based Nanomaterials for Bioimaging
Large surface area and feasible further functionalization make graphene-based nanomaterials a promising candidate for biomedical applications. However, as a result of their chemical sturctures, graphene nanosheets lack photoluminescence and rGO only display weak fluorescence, which makes it difficult to be utilized in bioimgaing applications. Many researchers attempted to resolve this problem by conjugation of fluorescent dyes and probes onto the large surface of graphene and its derivatives. Sun et al. reported an assembly strategy to prepare fluorescence probe RACD functionalized a single layer GO via π-π interaction and hydrogen bonding. The resluting nanomaterials exhibited that the fluorescent probes reduce the aggregation degree and acquire very well monodispersion, hydrophilicity and photostability, which is attributed to the strong synergy between RACD and GO [102]. Even so, fluorescence quenching remains a critical issue for these materials. In addition, the biocompatibility and toxicity of polymers applied to connect graphene-based materials and fluorescent moieties have not been adequately investigated. These facts appeal for alternative solutions to utilize graphene and its derivatives in bioimaging applications. Georgia et al. developed intrinsically photoluminescent graphene derivatives that show desirable biocompatibility and tunable fluorescence properties [103]. They can be organophilic or hydrophilic with different amine functionalization dodecylamine and hexamethylenediamine, respectively. The intrinsic fluorescent graphene-based nanomaterials possess great potential in a variety fileds of bioimgaing.
Metal Nanomaterials for Bioimaging
In recent years, fluorescent metal nanoparticles have shown great potential in bioimaging for improved disease diagnosis and treatment [104]. Gold is the most commonly used metal for bioimaging. The surface of AuNPs can be easily modified with various biomolecules such as peptides, proteins, antibodies, enzymes, and nucleic acids. These biomolecules can interact with specific cells or organelles in vivo, which makes it possible for AuNPs to be used for targeted optical imaging. Gao et al. reported a real-time in situ imaging of nucleus by AuNPs fabricated with bifunctional peptides constructed with both Au-binding affinity and nucleus-targeting ability. The bifunctional peptides showed strong binding affinity toward AuNPs and ensured good surface coverage of the nanoparticles, which made it stable and efficient for precise bioimaging of the nucleus in cells [105]. The Au-Se bond is considered as a better candidate than the Au–S bond to link the peptides and AuNPs due to the stronger ability against interference of intracellular thiol. Pan et al. prepared the Au-Se-peptide nanoprobes through a direct freezing process. The obtained nanoprobe was successfully applied to identify autophagy and apoptosis in chemotherapeutic drug treated cancer cells [106].
As a novel fluorescent imaging technology, DNA-templated silver nanoclusters (DNA-Ag NCs) have aroused the attention of many scientists due to their unique properties, especially the tunable fluorescence emission range relying on DNA sequences. However, the highly negatively charged DNA backbones have always been a great obstacle for the expansive applications in bioimaging because of poor stability as well as poor cell permeability in physiological environment. It is also noteworthy that the PL property and fluorescent efficiency of DNA-Ag NCs are far from satisfying. As a result, figuring out how to neutralize the negative charge on the surface of DNA strands is of great urgency for researchers. Recently, Lyu and co-workers successfully modified fluorescent DNA-Ag NCs with cationic polyelectrolytes via electrostatic force between the positively charged polyelectrolytes and the negatively charged phosphate groups of the DNA strands, leading to a threefold fluorescence intensity enhancement [7] (Fig. 5). Li et al. reported a facile strategy to make gold nanoclusters with positive charge and silver nanoclusters with negative charge form aggregates by electrostatic interactions. An incredible 40-fold fluorescence intensity enhancement was obtained. Results demonstrated that the physiological stability improved a lot and the cell permeability was also enhanced, which promises its practical applications in the future.
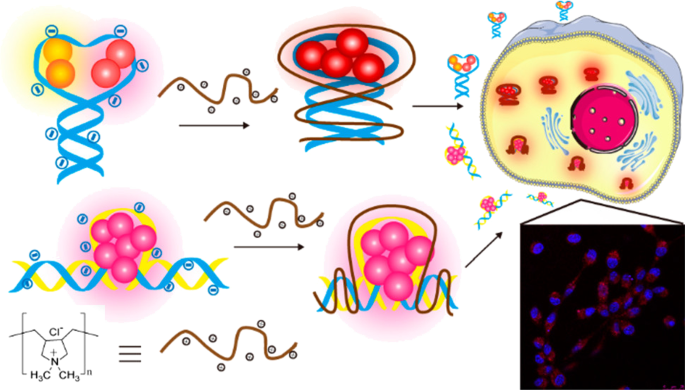
Formation of FL DNA–Ag NC–Cationic Polyelectrolyte Complexes for Cell Imaging [7]
Silica Nanoparticles for Bioimaging
Dye-doped fluorescent silica nanoparticles emerge with great potential for bioimaging as a novel and ideal platform for the monitoring of living cells and the whole body. The outer silica shell matrix protects fluorophores from outside chemical reaction factors as well as provides a hydrophilic shell for the inside insoluble nanoparticles, which renders the enhanced photo-stability and biocompatibility to the organic fluorescent dyes. Benefiting from the robust structure of silica matrices, dye-doped fluorescent silica nanoparticles have been presented with several superior properties including good biocompatibility, hydrophilic features, and high fluorescence intensity [107].
Jiao et al. also constructed a local hydrophobic cage in dye-doped fluorescent silica nanoparticles to improve their optical properties, which solves the problems of aggregation-caused quenching (ACQ) and poor photostability in aqueous media by organic fluorescence dyes benefiting from the robust structure of silica nanoparticles [108]. In addition, compared with free dyes, the fluorescent intensity both in water solution and living cells demonstrated a 12.3-fold enhancement due to the limitation of molecular motion, indicating a significant development for silica nanoparticles in biomedical applications. QDs have been developed for bioimaging both in vivo and vitro owing to their excellent optical qualities. However, a critical obstacle faced in QDs’ application in vivo is their poor biocompatibility. Inspired by the organic dye-conjugated silica-NPs, QDs-embedded silica-NPs have also been invented with the advantage that the excellent optical qualities of QDs can be retained, while the silica-NPs coat improves their biocompatibility to a large extent simultaneously. Darwish et al. reported that many QDs could be assembled around a central silica nanoparticle to form supra-NP assemblies. It was expected to be used for enhanced bioimaging because of their higher sensitivity and superior signal-to-background ratios [109]. There is reason to believe that silica-NPs conjugated with fluorescent nanomaterials with ideal optical properties will still be the dominant research interest in the future.
Phosphors for Bioimaging
For bioimaging, the sizes of phosphors need to be controlled so that they are small enough to be integrated with living cells. Furthermore, the aggregation of particles should be avoided for biocompatibility. Hence, the control of both particle sizes and dispersity in an aqueous solution is essential for the bioimaging application of the phosphors. Atabaev et al. prepared Eu, Gd-codoped Y2 O3 phosphors which had a spherical morphology within the range 61–69 nm. Enhanced PL emission and low toxicity made these phosphors suitable for bioimgaing applications [110].
Upconversion nanomaterials are able to convert lower-energy near-infrared photons to higher-energy ones as emission. This anti-Stokes photoluminescence process will lead to low background noise, large tissue penetration depth, and low photo-damage in bioimaging applications [111]. Lanthanide-based phosphors are able to show upconversion emission owing to their photodurability and low phonon energy. Nallusamy et al. reported a NIR–NIR bioimaging system based on Er 3+ :Y2 O3 phosphors by using NIR emission at 1550 nm under 980 nm excitation, which can allow a deeper penetration depth into biological tissues than ultraviolet or visible light excitation [112]. In addition, the surface of Er 3+ :Y2 O3 was electrostatically PEGylated to improve the chemical durability and dispersion stability under physiological conditions. Thakur et al. synthesized Ho 3+ /Yb 3+ co-doped GdVO4 phosphors via a modified sol–gel method. The prepared phosphors showed brilliant red upconversion emission under NIR excitation, which may be useful in bioimaging of the biomolecules [113].
Organic Frameworks for Bioimaging
Careful selection of MOF constituents can yield crystals of ultrahigh porosity and high thermal and chemical stability, with some of them being luminescent [114]. Recently, Sava Gallis’s group described a novel multifunctional MOF material platform which showed a wide spectral region from 614 to 1350 nm covering the deep red to NIR region. Both porosity and tunable emission properties made them highly suitable for in vivo bioimaging [115]. What’s more, to overcome the obstacle of MOF’s low selectivity towards malignant tissues, Liu et al. developed a target-induced bioimaging by conjugating DNA aptamers using ZrMOF nanoparticles as quenchers [116]. Based on the quenching of ZrMOF nanoparticles, target-induced bioimaging is achieved upon binding with the target.
Biodetection
Since fluorescent nanomaterials can amplify the fluorescent signals significantly and be compatible with organisms, there is increasingly more research on their application in the rapid detection of biomolecules [117]. It will shorten the analysis time to a large extent if we are able to establish a real-time detection system by fluorescent nanomaterials. It has been discovered that multiple detection can be achieved by using QDs probes simultaneously [118, 119].
Pathogen Detection
Pathogens have been an unignorable threat to human health for centuries and these include many types of microorganisms ranging from bacteria (pathogenic Escherichia coli, Salmonella, and Streptococcus pneumoniae ) and viruses (Coronavirus, Influenza virus and hepatitis virus ) However, conventional methods for pathogen detection still need improvement of detection limits and detection speeds. For their applications in detecting pathogens, Tan and co-workers reported a bioconjugated nanoparticle-based biodetection for in situ pathogen quantification, which cost less than 20 min [120]. Tan’s success promises that quick and convenient pathogen detection is possible and can be achieved with these ingenious nanomaterials in the future. Here, we list diverse pathogens detected by fluorescent nanomaterials as shown in Table 2 [119, 121,122,123,124,125,126,127,128,129].
Nucleic Acid Detection
Apart from pathogen detection, fluorescent nanomaterials have also aroused more and more interest of scientists in the detection of DNA. Owing to the merits that a number of biomolecules can be attached to the surface of fluorescent nanomaterials, the signal intensity of fluorescent nanomaterials in DNA detection can be enhanced significantly. Tan and co-workers developed an DNA detection method to detect gene products using bioconjugated dye-doped fluorescent silica nanoparticle with high sensitivity and photostability [130]. Although the analysis of nucleic acid has been successfully achieved by real-time nanomaterial fluorescence systems, there are still many shortcomings such as complex procedures or expensive instruments. To address these disadvantages, Wang’s group introduced a highly sensitive and visualized detection of nucleic acid by the combination of strand exchange amplification (SEA) and lateral flow assay strip (LFA) [131]. The system, which is possible to be widely used in areas requiring limited resource, is mainly characterized by integrating SEA with LFA (Fig. 6). There is no denying that the extremely high fluorescent signal for bioanalysis plays an irreplaceable role in these applications.
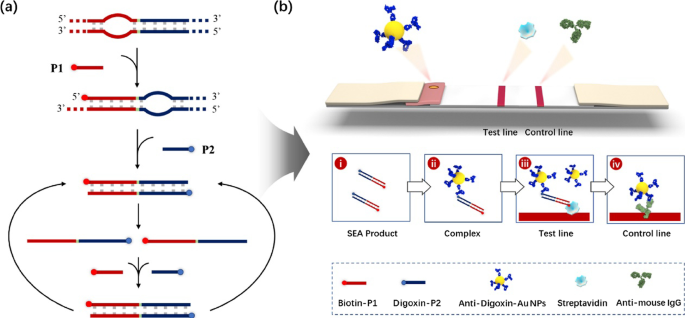
Schematic illustration of the SEA-LFA strip for the detection of nucleic acids [110]
Drug Detection
In the field of drug analysis, a facile and low-cost analytical method is always in demand for high-speed detection of specific pharmaceutical compounds. Real-time detection of drugs can be achieved with selective and sensitive fluorescent nanomaterials owing to their outstanding optical properties. In the past decade, the modification of nanomaterials has lowered their detection limit and improved their detection accuracy significantly. Recently, it is reported that ampicillin can be detected in serum sample based on aptamer, its complementary strand (CS) and gold nanoparticles (AuNPs) [132]. The limit of detection (LOD) of this method can be as low as 29.2 pM. However, there are still many limitations in the detection of drugs or target molecules in vivo. Due to low selectivity, conventional fluorescent nanomaterials inevitably generate false positive results and adverse effects in vivo. In addition, current tracking systems can hardly realize real-time tracking because of insufficient labels and excitation sources. Considering the above limitations, a new method using up/down conversion (UC/DC) PL nanomaterials has attracted increasing attention. Seo et al. reported a single-photon-driven UC/DC system which demonstrated outstanding performance in the detection of heavy metal ion (i.e. Hg 2+ ) in mussels [133]. LOD of the nanohybrids was ca. 1 nM. This system is appealing to researchers in the field of fluorescent nanomaterials for biomedical applications.
Drug Delivery
Until now, the technology of treating cancers with high efficiency and targeting function is not perfect enough. Under most circumstances, the anticancer drugs are distributed and released extensively in the body, which endangers the healthy cells and tissues irreversibly. Currently, a large variety of carriers for drug delivery have been designed. However, we can hardly supervise the distribution and result of the whole delivery process. Benefiting from the recent development of the surface modification technique, fluorescent materials capped with polymers like polyethylene glycol (PEG) can bond with drugs strongly and firmly. Then, the loaded drugs will be released in response to certain conditions such as pH, osmotic gradient and the surrounding environment. However, it should be confirmed whether the drugs are transported to the specific site or not. It’s also necessary for us to consider more details such as how much of the drugs is released in different positions. Aside from being drug carriers, fluorescent nanomaterials can also demonstrate the consequences of intracellular uptake due to their fluorescence property. QDs have been applied to monitor some important properties, such as delivery efficiency, release rate and distribution of drug molecules in vivo, which are beneficial for scientists in order to understand the specific targeting pathways of drug delivery within living cells. Duan and co-workers reported a facile pH-responsive fluorescent CDs drug delivery system [134]. Loaded with dox which is effective for gastric cancer, intracellular drug delivery and tracking could be simultaneously realized in patients (Fig. 7). The report highlighted the ability of fluorescent CDs to label and track the drug delivery process for at least 48 h, which showed a great potential in bioimaging, biolabeling and traceable drug delivery. Duan et al. designed a pH and receptor dual-responsive drug delivery system [135]. Hyaluronic acid was covalently attached to the surface of CDs, and doxorubicin was loaded by electrostatic self-assembly. In the tumor microenvironment (pH 5.6), the drug is released rapidly from the drug delivery system, while in the normal physiological environment (pH 7.4), the drug is hardly released. Endocytosis occurs when the drug delivery system reaches CD44 which is a receptor rich in tumor cells and can bind specifically to the hyaluronic acid. In addition, carbon nanotubes can be used for drug delivery by virtue of their high loading efficiency. Strong π-π interactions play a critical role in binding therapeutic agents with carbon nanotubes, which can be broke through changing external conditions, resulting in the release of drugs in specific position. Pennetta et al. functionalized single and multi-walled carbon nanotubes with a pyrrole derived compound to form a doxorubicin stacked drug delivery system. Biological studies showed that the synthesized nano-conveyors can effectively deliver the drug into cell lines and improve the therapeutic effects of doxorubicin [136].
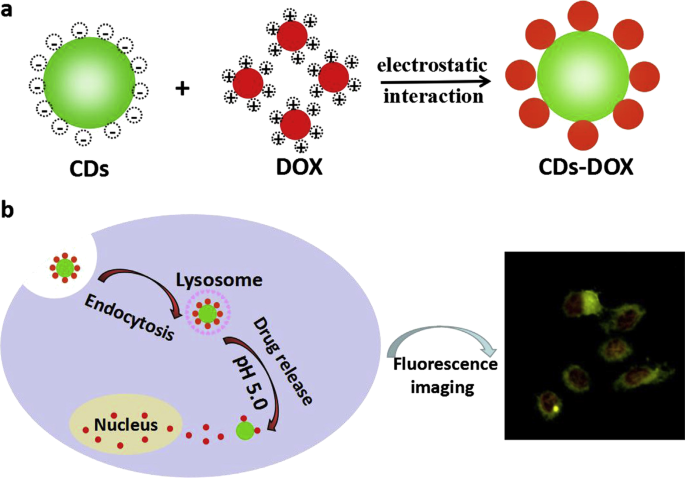
Schematic illustration of the preparation (a ) and cellular uptake (b ) of the CDs-DOX drug delivery system [113]
Photodynamic Therapy
Photodynamic therapy (PDT) is a novel therapy method for tumors which utilizes the interaction between light and photosensitizer. In PDT, reactive oxygen species (ROS) is produced from oxygen by photosensitizers in the condition of specific wavelengths of light (mostly in the area of near infrared light). The specific mechanism is presented in Fig. 8. ROS includes singlet oxygen, superoxide radicals, hydrogen peroxide, and hydroxyl radicals that possess strong cytotoxicity which cause significant destruction of tumor cells. However, there exist many defects such as limited penetration depth [137], hydrophobic properties [138], photobleaching [139], complicated procedure [140], and tumor hypoxia. PDT agents can hardly be dissolved and they will disperse extensively in vivo once they are taken, making it impossible to be targeted and selected. Fluorescent nanomaterial based photodynamic therapy developed fast in recent years [141]. Combined with the unique properties that QDs possess, such as high fluorescent efficiency and great spectral resolution, the effect of PDT can be enhanced. Barberi-Heyob, M and coworkers significantly enhanced the photodynamic efficiency with a concentration of 8 nM because of the light dose-dependent response [142]. In addition, photodynamic therapy can sometimes do harm to the skin and eyes of patients due to its photosensitive side-effect. To alleviate these adverse effects, a novel nanoparticle-based drug carrier for photodynamic therapy is reported which can provide stable aqueous dispersion of hydrophobic photosensitizers. Meanwhile, the key step of photogeneration of singlet oxygen was preserved, which is necessary for photodynamic action [143]. It is obvious that QDs combined photodynamic therapy will replace the conventional PDT someday.
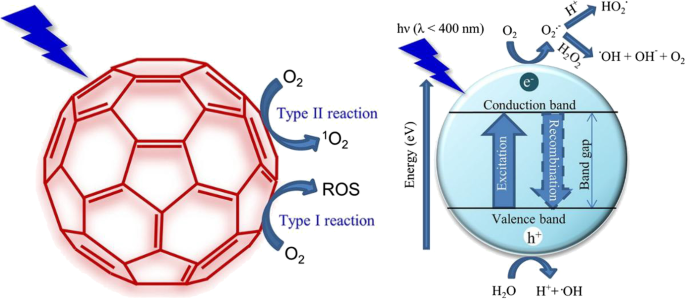
Schematic illustration of producing reactive oxygen species (ROS) for the photodynamic therapy (PDT) [119]
Challenges
Synthesis Challenges
Achieving Uniform Distribution
In the synthesis process, the diameter and size distributions of FNMs can be hardly distributed uniformly due to the agglomeration of small particles. This could be fatal to the optical properties of FNMs in biomedical application. For this reason, the applications of FNMs are still at the laboratory scale. It has been confirmed that the surface properties primarily determine the agglomeration state of the nanoparticles and their size. Therefore, surface modification is promising to achieve uniform distribution of FNMs by altering their surface properties [144]. To date, silanized QDs have been widely used because the polymerized silica coating increases the stability in buffers under physiological conditions [145]. Carbon dots synthesized by hydrothermal reaction using water-soluble base were reported to be difficult to control the size and distribution of grain boundary [146]. Khanam et al. reported a facile and novel synthetic method for the preparation of hydroxyl capped CDs using an organic base and a surfactant (Triton X-100) to modify the surface. A narrow particle size distribution at 7.2 nm was found in Raman and DLS studies, which is smaller than the majority of the particles falling within the range of below 10 nm in diameter [147].
Fluorescence Quantum Yield
Fluorescence quantum yield plays a crucial role for FNMs in their efficiency for on-demand light emission. Tunable and highly fluorescent CDs can be prepared with the surface functionalization approach. Nitrogen-doped FNMs are reported to have improved fluorescence quantum yield. With increasing nitrogen content, fluorescence quantum yield can be increased to as high as 56% at high synthesis temperature [148]. A facile strategy was also developed to tune the photoluminescent properties of CDs using a microwave irradiation, with citric acid and nitrogen-containing branched polyethyleneimine (b-PEI) as precursors. At intermediate levels of b-PEI, the CDs produced a high photoluminescence yield [149]. Lin et al. explored carbon dots with a high-fluorescence quantum yield rate synthesized from L-cysteine and citric acid by the microwave-assisted method. The obtained carbon dots exhibited a high-fluorescence quantum yield (up to 85%), which is due to the combination of amidogens and sulfydryl with carbon dots, and henceforth bringing the improved fluorescence property [150]. The above examples demonstrate that nitrogen or other electron-rich atoms like sulphur can obtain satisfying fluorescence quantum yield.
Aggregation-Caused Quenching
Fluorescent molecules can emit light with high efficiency in dilute solution. However, in concentrated solution or solid state, their fluorescence will be weakened or even disappear. This phenomenon is called Aggregation-Caused Quenching (ACQ) [151]. This problem has been puzzling scientists for almost 150 years, thus hindered the extensive application of fluorescent dyes.
In order to make effective use of fluorescent dyes, scientists have attempted many methods. Most of them focused on reducing the concentration of fluorescent dyes to prevent ACQ effect. Tang et al. discovered the phenomenon of Aggregation-Induced Emission (AIE) [152]. Based on rationally designed molecules, the fluorescence of organic molecules in solid state can be attained. Still, for more than one hundred thousand different fluorescent dyes in the world, the problem of ACQ has not been completely resolved. As long as they aggregate together, ACQ will make them lose their fluorescent properties.
It is almost impossible for high concentration or solid state FNMs to show reliable fluorescence due to fluorescence quenching. Although fluorescence quenching can be used as a sensitive signal to indicate substrate concentration in analytical chemistry, [153] in the most circumstances, however, fluorescence quenching is undesirable for FNMs because it always has considerable influence on bioimaging and biodetection. To overcome this long-standing problem, Benson et al. reported a universal solution with the discovery of a class of materials called small-molecule ionic isolation lattices (SMILES) [154]. SMILES are simple to make by mixing cationic dyes with anion-binding cyanostar macrocycles. We draw inspiration from their findings and believe that similar results can be obtained if we replace cationic dyes with cationic modified FNMs.
Application Challenges
Drawbacks of UV Light FNMs
Although FNMs realized the great-leap-forward from in vitro imaging to in vivo imaging, the emission fluorescence of most of FNMs is distributed in ultraviolet region or short wavelength visible region, which limits the optical imaging in living organisms. Moreover, use of UV light for monitoring living processes in cells and tissues has some potential drawbacks as long‐term irradiation of living cells may cause DNA damage and cell death. Therefore, the development of FNMs in near-infrared region is urgently needed in the future. Although NIR FNMs have deep tissue penetration, NIR detectors and filters are needed as the excitation and emission wavelengths are too close to each other, which restricts their range of application.
Interference in Biological Environment
Almost all biological tissues will produce significant autofluorescence under short wavelength, UV and visible light radiation [155]. Autofluorescence reduces the signal‐to‐background ratio and often interferes seriously with the visual effects. Some substances in the substrate of biological tissues also have great influence on the fluorescence, which reduces the selectivity of FNMs significantly. Until now, although the application of FNMs in mice showed acceptable outcomes, it is still difficult to achieve similar results in larger mammals. Much higher luminous efficiency under low power density excitation is required to avoid the background signal interference. Furthermore, temperature and pH conditions of the biological environments strongly affect the fluorescence of some substances as well. Therefore, satisfying fluorescence of FNMs at 37 °C and physiological pH should be guaranteed. It's worth noting that the pH in tumor is lower than normal tissues. Hence, fluorescence with high selectivity in acid environment will improve the efficiency of FNMs.
Biocompatibility
Biocompatibility refers to materials or systems that are nontoxic, safe and not causing physiological or immunological reactions. QDs with unique quantum confinement effect and electro-optical properties are attractive for biomedical applications. However, toxic effects of traditional semiconductor QDs made of heavy metal ions have serious safety concerns for their undesired environmental or health effects. In the purpose of circumventing this problem, core–shell structure modification of QDs by using biocompatible ligands or polymers is one way to effectively minimize toxic effects of traditional QDs. Furthermore, scientists are searching for heavy metal-free QDs formulations. Non-toxic or less toxic carbon dots and silica nanoparticles have shown their potential as the ideal FNMs for biomedical applications. Impurities brought in the process of syntheses may influence the biocompatibility of fluorescent nanomaterials. In order to reduce the influence of toxic impurities, green synthesis methods have been arousing the interest in biomedical fields. Chowdhury et al. utilized cacao extract which is a natural product as a reducing and stabilizing agent in the synthesis of gold nanoparticles [156]. Oxalic acid, as a constituent of cacao, can reduce Au 3+ in HAuCl4 to metallic gold and stabilize the resultant nanoparticle colloidal solution. In vitro studies suggested that the cacao derived gold nanoparticles are biocompatible and suitable for biomedical applications. For MOFs, appropriate metal ions and ligands must be selected to lower the toxicity. Wang et al. employed Fe, Ti and Zr as constituents of MOFs, which are harmless and even beneficial elements to the body [157]. In vitro studies indicate that the proposed material has good biocompatibility and safety in biomedical application. What’s more, it is necessary to consider whether the difference in composition, surface charge, or modified group will have different biological effects. Taking these factors into account, we can improve the biocompatibility of FNMs with rational design.
Conclusions
Benefiting from the unique properties of fluorescent nanomaterials, some limitations and barriers of conventional materials and methods in biomedical applications can be broken through. In this review, we comprehensively present the synthesis methods and applications of fluorescent nanomaterials. The advanced synthesis methods can offer us the fluorescent nanomaterials with ideal morphology, size ranges and structures. Meanwhile, the more convenient syntheses can lower the manufacturing cost of fluorescent nanomaterials, which is critical to their widespread applications in biomedical fields. Based on the improved synthesis techniques, the performance of fluorescent nanomaterials is bound to leap in their applications. With the development of fluorescent nanomaterials, bioimaging, biodection, drug delivery and photodynamic therapy will be more widely applied in the diagnosis and treatment of diseases. Finally, challenges in synthesis and biomedical applications point out exiting questions and developing direction. We hope that this review can bring some new insights to the development of fluorescent nanomaterials.
Availability of data and materials
All data and materials are available without restrictions.
Abbreviations
- FNMs:
-
Fluorescent nanomaterials
- PL:
-
Photoluminescence
- CDs:
-
Carbon dots
- QDs:
-
Quantum dots
- b-PEI:
-
Branched polyethyleneimine
- SWCNTs:
-
Single-walled carbon nanotubes
- CQDs:
-
Carbon quantum dots
- GQDs:
-
Graphene quantum dots
- NCQDs:
-
Nitrogen-doped carbon quantum dots
- NSCDs:
-
Nitrogen and sulfur doped carbon dots
- QY:
-
Quantum yield
- MCDs:
-
Multi-doped carbon dots
- MWCHTs:
-
Multi-walled carbon nanotubes
- GO:
-
Graphene oxide
- rGO:
-
Reduced graphene oxide
- CVD:
-
Chemical vapor deposition
- AuNPs:
-
Gold nanoparticles
- Ag NCs:
-
Ag nanoclusters
- Cu NCs:
-
Cu nanoclusters
- NPs:
-
Nanoparticles
- TEOS:
-
Tetraethylorthosilicate
- MR:
-
Magnetic resonance
- COFs:
-
Covalent-organic frameworks
- TNP:
-
2,4,6-Trinitrophenol
- MOFs:
-
Metal organic frameworks
- ACQ:
-
Aggregation-caused quenching
- NIR:
-
Near infrared
- SEA:
-
Strand exchange amplification
- LFA:
-
Lateral flow assay strip
- CS:
-
Complementary strand
- LOD:
-
Limit of detection
- UC:
-
Upconversion
- DC:
-
Downconversion
- PDT:
-
Photodynamic therapy
- ROS:
-
Reactive oxygen species
- AIE:
-
Aggregation-induced emission
- SMILES:
-
Small-molecule ionic isolation lattices
Nanomateriais
- Sensores e processadores convergem para aplicações industriais
- O que vem a seguir para a impressão 3D - Aplicações Biomédicas
- Os cinco principais problemas e desafios para 5G
- Nanopartículas para terapia do câncer:progresso e desafios atuais
- Compostos de grafeno e polímero para aplicações de supercapacitor:uma revisão
- Últimos avanços e aplicativos na tecnologia IoT
- A EconCore licencia a Fynotej para aplicações automotivas de NA e avança em termoplásticos de alto desempenho (HPT)
- Acionamentos para aplicações de refrigeração industrial e refrigeração industrial
- Tornos giratórios para aplicações de mandrilamento e torneamento
- Compreendendo os benefícios e desafios da fabricação híbrida