Nanopartículas multifuncionais de ouro para aplicações diagnósticas e terapêuticas aprimoradas:uma revisão
Resumo
As propriedades médicas dos metais foram exploradas durante séculos na medicina tradicional para o tratamento de infecções e doenças e ainda praticadas até hoje. Os medicamentos à base de platina são a primeira classe de medicamentos à base de metal a serem usados clinicamente como agentes anticâncer, após a aprovação da cisplatina pela Food and Drug Administration (FDA) dos Estados Unidos há mais de 40 anos. Desde então, mais metais com benefícios para a saúde foram aprovados para ensaios clínicos. Curiosamente, quando esses metais são reduzidos a nanopartículas metálicas, eles exibem propriedades únicas e inovadoras, superiores às de suas contrapartes em massa. Nanopartículas de ouro (AuNPs) estão entre as nanopartículas metálicas aprovadas pelo FDA e têm se mostrado uma grande promessa em uma variedade de funções na medicina. Eles foram usados como agentes de liberação de drogas, fototérmicos (PT), de contraste, terapêuticos, de radiossensibilização e de transfecção de genes. Suas aplicações biomédicas são revisadas aqui, cobrindo seu uso potencial no diagnóstico e terapia de doenças. Alguns dos sistemas baseados em AuNP que são aprovados para ensaios clínicos também são discutidos, bem como as ameaças potenciais à saúde de AuNPs e algumas estratégias que podem ser usadas para melhorar sua biocompatibilidade. Os estudos revisados oferecem uma prova de princípio de que os sistemas baseados em AuNP podem ser usados sozinhos ou em combinação com os sistemas convencionais para melhorar sua eficácia.
Introdução
A medicina está entre os muitos campos que se beneficiaram com a nanotecnologia. A nanotecnologia surgiu com muitas oportunidades para melhorar e desenvolver novos agentes diagnósticos e terapêuticos por meio do uso de nanomateriais [1, 2]. AuNPs, em particular, exibem propriedades físico-químicas únicas e boa estabilidade química. Eles são fáceis de funcionalizar com quase todos os tipos de moléculas doadoras de elétrons, por meio de várias químicas ou com base em sua forte afinidade por moléculas tioladas [3, 4]. Devido ao seu tamanho minúsculo, AuNPs têm uma área de superfície maior e alta capacidade de carga de drogas. Múltiplas frações podem ser incorporadas nos AuNPs para aplicações biomédicas; estes incluem moléculas de direcionamento para aumentar a especificidade, agentes de contraste para bioimagem e para monitorar a resposta da doença aos medicamentos em tempo real, e agentes terapêuticos para o tratamento da doença [5, 6]. Curiosamente, mesmo sem quaisquer biomoléculas adicionadas, AuNPs são capazes de direcionamento, imagem e tratamento de doenças. Com base em suas propriedades dependentes de tamanho, novos sistemas baseados em AuNP podem ser criados para uso em várias aplicações biomédicas [7].
AuNPs são feitos de um precursor de metal que é termoestável e, portanto, muito estável e não biodegradável. O ouro a granel é usado na medicina e provou ser bio-inerte e não tóxico [8, 9]; portanto, o núcleo de ouro nos AuNPs irá essencialmente exibir propriedades semelhantes [3, 10]. AuNPs e suas aplicações foram extensivamente estudados por mais de cinco décadas e mostraram grande promessa como agentes teranósticos em estudos pré-clínicos [5, 11,12,13] e clínicos [14,15,16,17,18]. Existem muitas outras oportunidades para novos sistemas baseados em AuNP, conforme discutido nesta revisão. AuNPs já são explorados em ensaios clínicos como carreadores de drogas para o tratamento de cânceres em estágio avançado [16, 17], e como agentes PT no tratamento de câncer de próstata [19] e acne [18]. Sem prejudicar a saúde e as questões regulatórias que cercam o uso de AuNPs [20], um futuro para esses sistemas em biomedicina está próximo. Sistemas multifuncionais baseados em AuNP que são capazes de combater a resistência aos medicamentos com eficácia localizada e melhorada são possíveis [11, 21, 22]. A revisão destaca as propriedades biológicas dos AuNPs em estudos pré-clínicos e clínicos, refletindo sobre suas bioaplicações como agentes diagnósticos e terapêuticos. Suas ameaças potenciais à saúde e estratégias que foram usadas para superar suas limitações também são descritas. Finalmente, as perspectivas futuras das AuNPs na medicina são destacadas.
Nanopartículas de ouro
A popularidade dos AuNPs em aplicações médicas ganhou muito impulso devido às suas propriedades físicas e químicas exclusivas. AuNPs são partículas coloidais sólidas que variam em tamanho de 1 a 100 nm [23]. As aplicações de AuNPs em biologia estão enraizadas em suas propriedades físico-químicas, não se limitando ao seu tamanho, ressonância plasmônica de superfície (SPR), forma e química de superfície [3, 10]. Esses parâmetros influenciam sua atividade e os tornam candidatos perfeitos para uso no diagnóstico e tratamento de doenças, seja como agentes de liberação, sensibilização, contrastes ou terapêuticos. Seu pequeno tamanho está associado a uma área de superfície maior, que permite a modificação da superfície e fixação de múltiplas cargas úteis, como direcionamento, imagem e agentes terapêuticos [4, 24,25,26]. Seu pequeno tamanho também possibilita que os NPs e sua carga atravessem barreiras biológicas que de outra forma seriam difíceis de alcançar e penetrar [11].
AuNPs estão sendo cada vez mais reconhecidos como agentes diagnósticos, terapêuticos e teranósticos (um agente que pode ser usado simultaneamente para diagnosticar e tratar uma doença), que têm potencial para abordar os efeitos fora do alvo associados às terapias convencionais. No entanto, AuNPs possuem propriedades e funções diferentes em comparação com suas contrapartes em massa biocompatíveis, o que pode ser perigoso para a saúde humana [27,28,29]. O uso clínico de compostos de ouro a granel para o tratamento de doenças é uma prática antiga e certificada como segura [8]. Nos últimos anos, a pesquisa mostrou que AuNPs têm propriedades médicas semelhantes ou melhoradas [29]. Devido às suas propriedades ópticas, químicas e físicas únicas, AuNPs frequentemente apresentam propriedades novas em comparação com o ouro em massa [30, 31] e podem servir como agentes diagnósticos e terapêuticos [5].
Síntese de AuNPs
AuNPs podem ser produzidos de várias maneiras, seguindo a abordagem de cima para baixo ou de baixo para cima. A abordagem de cima para baixo usa métodos físicos e químicos para produzir os tamanhos desejados do material a granel, enquanto a abordagem de baixo para cima envolve métodos químicos para montar os blocos de construção na formação de sistemas nanométricos [32, 33]. Os métodos físicos (como moagem, fotoquímica, radiação e litografia) usam muita energia e pressão para reduzir os materiais a granel em 10 –9 bilionésimo de um metro de tamanho [10, 32, 34]. Os processos de nucleação são facilmente controlados com o uso de métodos físicos, não sendo necessários agentes redutores, e com alguns desses métodos a síntese ocorre simultaneamente com a esterilização dos NPs. No entanto, as tecnologias físicas costumam ser caras, não estão prontamente disponíveis e requerem equipamento especializado. Além disso, os agentes de nivelamento e estabilização podem não sobreviver aos processos de alta energia envolvidos nesses processos [34].
A abordagem bottom-up é mais preferida na síntese de AuNPs, pois é rápida, fácil e não requer o uso de equipamentos sofisticados [33,34,35]. É baseado no método químico desenvolvido por Turkevich em 1951 (Fig. 1A), que usa citrato para redução e estabilização de um precursor de ouro, resultando na produção de AuNPs esféricos de 15 nm [3, 10, 23, 33, 36 , 37]. O método foi modificado pela variação da proporção de citrato para conteúdo de precursor de ouro e resultou em uma faixa de diâmetro de tamanho de 15-150 nm AuNPs (Fig. 1B) [10, 24]. Vários agentes redutores, como boro-hidreto de sódio, brometo de cetiltrimetilamônio (CTAB) e ácido ascórbico também foram introduzidos. Alguns dos agentes redutores químicos são infelizmente tóxicos [33, 34, 36] e geralmente passivados pela adição de agentes estabilizadores em sua superfície, como polietilenoglicol (PEG), goma arábica, polissacarídeos e peptídeos bioativos [37, 38].
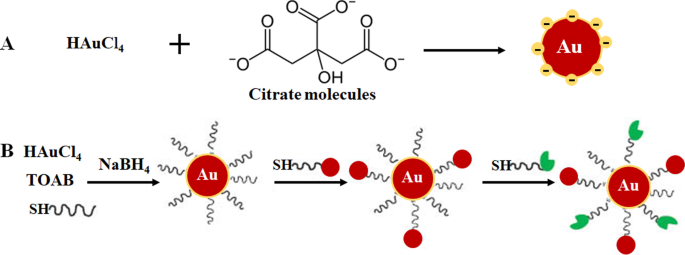
Formulação de AuNP através de sistema monofásico por redução de citrato ( A ) e redução do sistema de duas fases seguida de estabilização e funcionalização via reação de troca de ligante, método de Brust-Schiffrin ( B ) Reproduzido com permissão [36]. Copyright 2013, De Gruyter. TOAB brometo de tetrabutilamônio, SH moléculas tioladas
Abordagens mais ecológicas, como processo de plasma em líquido induzido por microondas (MWPLP) e nanotecnologia verde, têm sido exploradas em AuNPs de síntese para evitar o uso de agentes redutores químicos tóxicos. O MWPLP usa microondas para gerar nucleação de NPs metálicos e não requer nenhum agente redutor, e a energia necessária para a síntese é muito baixa [34]. A nanotecnologia verde, por outro lado, utiliza compostos naturais provenientes de plantas e microrganismos como fonte de agentes redutores na síntese de AuNPs biogênicos [12, 33, 39,40,41]. A nanotecnologia verde é considerada ecologicamente correta e, portanto, mais adequada para aplicações biomédicas. A síntese mediada por plantas é mais econômica do que o uso de microorganismos. Além disso, a síntese pode ser realizada em apenas uma etapa, e os NPs são mais fáceis de purificar. Além disso, as plantas são renováveis; várias partes das plantas, como folhas, caules, cascas, raízes, flores e frutos podem ser colhidos sem matar a planta e usados para síntese. Os extratos preparados a partir do material vegetal contêm fitoquímicos, proteínas e enzimas que podem funcionar como agentes redutores, estabilizadores e capeadores [10, 12, 24, 34, 35, 40, 42]. A epigalocatequina dos chás verdes [42] e a mangiferina (MGF) das mangas [12, 43] estão entre os compostos derivados de plantas que foram amplamente usados para sintetizar AuNPs [34]. Mais informações sobre esses métodos são revisadas extensivamente nas referências a seguir [10, 24, 34, 35].
Aplicação biológica de AuNPs
O papel e a importância dos AuNPs na ciência médica estão, sem dúvida, se tornando mais visíveis, o que é apoiado pelo número crescente de estudos que demonstram sua aplicação multifacetada em uma ampla gama de campos biomédicos. A biocompatibilidade dos AuNPs é atribuída à longa história do ouro no tratamento de doenças humanas, que remonta a 2500–2600 aC. Os chineses e indianos usavam o ouro para o tratamento da impotência masculina, epilepsia, sífilis, doenças reumáticas e tuberculose. A China descobriu o efeito de longevidade do ouro coloidal vermelho, que ainda é praticado na Índia como parte da medicina ayurvédica para rejuvenescimento e revitalização. Cinábrio-ouro (também conhecido como Makaradhwaja) é usado para melhorar a fertilidade na Índia. Nos países ocidentais, o ouro tem sido usado para tratar distúrbios nervosos e epilepsia. Nenhuma toxicidade foi relatada para seu uso em estudos in vitro e in vivo [8, 44, 45]. Desde então, os compostos de ouro orais e injetáveis continuaram a ser usados como tratamentos para a artrite [9, 46] e também mostraram ter efeitos anticâncer [8]. Efeitos semelhantes e, em alguns casos, melhorados também foram relatados para AuNPs, que estão emergindo como agentes promissores para o diagnóstico de doenças [47,48,49] e terapia [3, 29, 50, 51].
AuNPs têm uma área de superfície maior que pode ser explorada para aplicações biomédicas, anexando várias biomoléculas para atender a uma função desejada. Estes podem incluir porções de direcionamento para ajudar a reconhecer biomarcadores específicos de doenças, agentes de contraste para bioimagem e agentes terapêuticos para o tratamento de doenças [24, 25]. A vantagem de usar AuNPs sobre outros nanomateriais é que eles podem ser facilmente funcionalizados usando vários produtos químicos, conforme demonstrado na Fig. 2 [4, 26]. AuNPs têm alta afinidade por moléculas tioladas, e a ligação tiol-ouro é o método mais comumente usado para adsorver moléculas na superfície NP [4]. Químicos baseados em afinidade, como ligação biotina-estreptavidina e acoplamento carbodiimida, também são usados. AuNPs são usados em três áreas principais da biomedicina:entrega de produtos farmacêuticos, fins diagnósticos e terapêuticos [24, 35], e têm demonstrado um enorme potencial nessas áreas, conforme discutido abaixo.
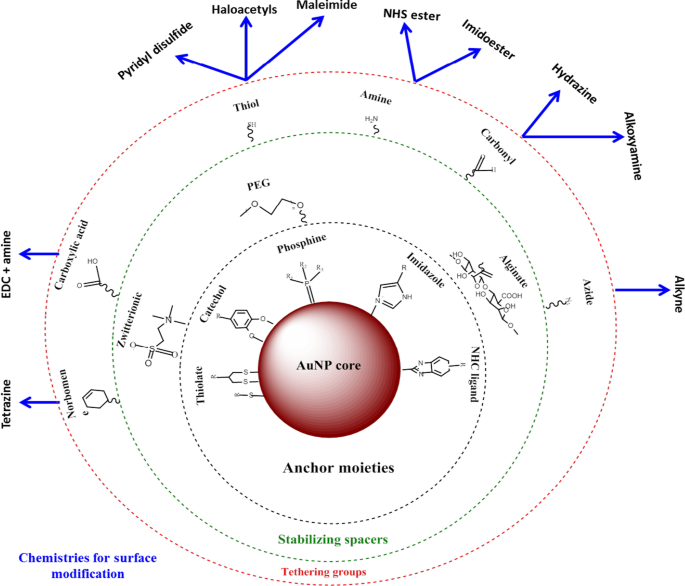
Síntese e funcionalização de AuNPs. Biomoléculas com grupos funcionais são primeiro adsorvidas na superfície NP através da afinidade ouro-tiol. Em seguida, outros grupos funcionais, como o grupo amina, podem ser usados para ligar moléculas com grupos carboxila para anexar o direcionamento ou frações de fármaco. Adaptado de [32]
AuNPs como agentes de entrega de medicamentos
A aplicação mais comum de AuNPs é como veículos de entrega de drogas [11, 18, 52], vacinas [53] e terapia gênica [24, 32]. AuNPs possuem propriedades que podem resolver a maioria dos problemas associados às terapias convencionais, como resistência a drogas, baixa distribuição de drogas, biodegradação e eliminação precoce de drogas [11]. AuNPs pode reduzir significativamente a dosagem do medicamento, a frequência do tratamento e é capaz de transportar medicamentos hidrofóbicos e insolúveis. Eles são considerados bioinertes e podem mascarar sua carga do ataque de células do sistema imunológico, proteger os medicamentos da degradação proteolítica conforme eles viajam pelo sistema circulatório e, assim, aumentar o tempo de circulação do medicamento. Esses fatores podem aumentar prontamente a eficácia dos medicamentos, concentrando-os e retendo-os nos tecidos doentes, com pouco ou nenhum efeito nos tecidos normais [25].
O uso de AuNPs no tratamento do câncer foi amplamente estudado [17, 37, 54] e, ao longo dos anos, foi estendido a outras doenças, como obesidade [50, 55, 56] e acne [18]. Os sistemas baseados em nanopartículas são menores do que a maioria dos componentes celulares e podem atravessar passivamente as barreiras celulares, aproveitando o efeito da permeabilidade e retenção aprimorada (EPR) na vasculatura dos tecidos doentes [25]. O EPR em um estado patológico é caracterizado por angiogênese excessiva e aumento da secreção de mediadores de permeabilidade, que podem aumentar a captação de AuNP pelos tecidos doentes. Essas características estão associadas apenas a estados patológicos e não a tecidos normais, o que fornece uma oportunidade para o direcionamento seletivo dos conjugados AuNP [25]. AuNPs são atraentes como transportadores de drogas, pois podem transportar várias moléculas simultaneamente, diversificando ainda mais suas propriedades. Esta é uma característica desejável na medicina em que a maioria das bioaplicações AuNP estão enraizadas, uma vez que AuNPs podem ser adaptados para uma função biomédica específica. Isso pode ajudar a controlar a maneira como eles interagem com organelas celulares e, portanto, é uma promessa para o desenvolvimento futuro de modalidades eficazes de diagnóstico e tratamento para várias doenças [4].
Sistemas de diagnóstico baseados em AuNP
O surgimento da nanotecnologia aumentou a posição no desenvolvimento de sistemas de detecção que são rápidos, robustos, sensíveis e altamente competitivos em comparação com os testes de diagnóstico convencionais [48]. Os nanomateriais são geralmente integrados em plataformas de biossensorização existentes para a detecção de marcadores de gases, DNA e proteínas envolvidos no desenvolvimento de doenças [47]. Entre os vários nanomateriais (que incluem NPs metálicos, poliméricos, magnéticos e semicondutores) usados em diagnósticos, AuNPs têm sido amplamente usados em biossensores, sensores eletroquímicos e ensaios cromogênicos para detectar ou sentir a presença de biomarcadores de doenças [49]. Seu SPR localizado (LSPR), transferência de energia de ressonância de fluorescência (FRET), espalhamento Raman aprimorado por superfície, condutividade, atividade redox e efeito de carga quantizado os tornam uma ferramenta ideal para geração de imagens e detecção de moléculas alvo [10, 24]. Suas propriedades eletrônicas e ópticas e capacidade de espalhar luz visível e infravermelha próxima (NIR) são compatíveis e mensuráveis com várias tecnologias, como técnicas microscópicas (espalhamento de luz de campo escuro, eletrônico e confocal) [57], tomografia computadorizada (TC) , Técnica de imagem PT heterodyne, espectroscopia UV-Vis e Raman [24, 35].
O desenvolvimento de sistemas de diagnóstico baseados em AuNP envolve a modificação da superfície de AuNP, por exemplo, por meio da fixação de biomoléculas que reconhecem biomarcadores de doenças [3, 24, 58]. Os ensaios de fluxo lateral (LFAs) são provavelmente o exemplo mais conhecido de ferramentas de diagnóstico baseadas em nanotecnologia. LFAs normalmente fazem uso de AuNPs de cerca de 30-40 nm porque partículas menores têm seções transversais de extinção muito pequenas, enquanto partículas maiores são geralmente instáveis para uso nesses ensaios [59]. Além disso, outras moléculas / enzimas que podem desencadear alterações em SPR, condutividade e redox de AuNPs estão incluídas. Esses indicadores fornecem um sinal detectável após a ligação dos analitos aos conjugados AuNP [24], a falta ou presença do sinal refletirá então a ausência ou presença da molécula alvo ou da doença. O sinal gerado por AuNPs é quimicamente estável, duradouro e consistente quando usado em diferentes formatos de teste:tubo de ensaio, tira, in vitro e in vivo [24]. Conseqüentemente, sua aplicação aumentou notavelmente a velocidade e o sucesso dos testes diagnósticos.
Ensaios Colorimétricos baseados em AuNP
Em ensaios colorimétricos, AuNPs produzem um sinal visual (geralmente uma mudança de cor) que pode ser detectado a olho nu, sem o uso de instrumentos avançados. Geralmente, uma solução coloidal de AuNPs tem uma cor de vermelho rubi a uva que é altamente dependente da distância entre as partículas [60, 61]. A ligação de um analito aos AuNPs modificados com elementos de bio-reconhecimento molecular (por exemplo, anticorpos, peptídeos, aptâmeros, enzimas, etc.) induz uma mudança distinta no LSPR, resultando consequentemente na mudança de cor de vermelho rubi para azul [60 , 62, 63]. A intensidade da cor é diretamente proporcional à concentração de um analito e usada para confirmar a presença e o estado da doença. O diagnóstico colorimétrico baseado em AuNP foi usado com sucesso na detecção do vírus influenza A [64], vírus Zika [65], T7 Bacteriophage [66], Mycobacterium tuberculosis [67], e recentemente, para a detecção de síndrome respiratória aguda grave-coronavírus-2 (SARS-CoV-2) [60, 68].
Um exemplo de um ensaio baseado em AuNP colorimétrico foi demonstrado para a detecção de SARS-CoV-2 [60], um vírus que causa uma doença viral altamente infecciosa Corona 2019 (COVID-19) [60, 68]. Com este ensaio, a presença do vírus foi relatada por uma simples mudança de cor; nenhuma instrumentação foi necessária para fazer o diagnóstico. Os testes de diagnóstico clínico atuais desse vírus usam o ensaio de reação em cadeia da polimerase em tempo real da transcriptase reversa (RT-PCR), que leva de 4 a 6 horas, enquanto os sistemas de ponto de atendimento rápido (PoC) detectam anticorpos que podem demorar vários dias para aparecer no sangue. Em comparação, o ensaio com base em AuNP colorimétrico foi mais robusto e mais rápido, conforme demonstrado na Fig. 3. A incubação de AuNPs marcados com oligonucleotídeos antisense (ASOs) na presença de amostras de ARN de SARS-CoV-2 resultou na formação de precipitado azul dentro ~ 10 min. Em um teste positivo para SARS-CoV-2, a ligação dos ASOs ao gene N na fosfoproteína do nucleocapsídeo do vírus induziu uma cor azul que foi detectada visualmente. O teste foi muito sensível e teve um limite de detecção de 0,18 ng / μL para o RNA SARS-CoV-2 [60].
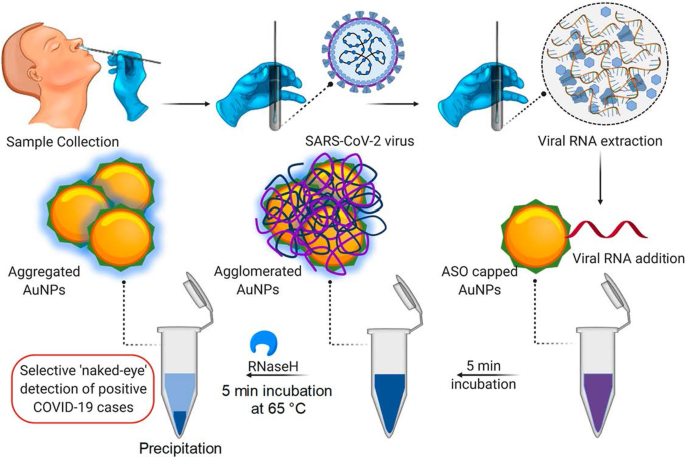
Sistema de diagnóstico colorimétrico baseado em AuNP. Detecção seletiva a olho nu de RNA de SARS-CoV-2 pelos AuNPs protegidos por ASO. Reproduzido com permissão [60]. Copyright 2020, ACS Nano
Os LFAs baseados em AuNP seguem o mesmo princípio que o mostrado na Fig. 3; entretanto, em vez de uma mudança de cor em uma solução, uma linha visível é formada em uma tira de teste quando um analito está presente. Na presença de um analito, AuNPs foram capturados na linha de teste e formaram uma linha vermelha distinta, que foi visualizada a olho nu. A intensidade da linha é determinada pelo número de AuNPs adsorvidos [69]. Um exemplo de um LFA simples e rápido baseado em AuNP é mostrado na Fig. 4, para a detecção de Pneumocystis jirovecii ( P. jirovecii ) Anticorpos IgM em soros humanos. Os AuNPs de 40 nm foram conjugados com os antígenos sintéticos recombinantes (RSA) de P. jirovecii , a principal glicoproteína de superfície ou serina protease semelhante a kexina, que foram usadas como um indicador para a presença ou ausência de P. jirovecii . Em um teste positivo, o P. jirovecii IgM foi capturado pelo conjugado AuNP-RSA na almofada de conjugado. O complexo AuNP-RSA / IgM então flui para a membrana analítica onde se liga ao IgM anti-humano (linha de teste) e o excesso se move para os anticorpos anti-RSA (linha de controle), resultando em duas linhas vermelhas. O teste negativo terá apenas uma cor vermelha na linha de controle [70]. Um estudo independente usou o LFA baseado em AuNP para detectar seletivamente o SARS-CoV-2 IgM como confirmado pelo aparecimento de linhas vermelhas em ambas as linhas de teste e controle [68]. A cor foi detectada visualmente a olho nu em 15 minutos nos dois sistemas, e apenas 10–20 μL de amostras de soro foram necessárias por teste [68, 70].
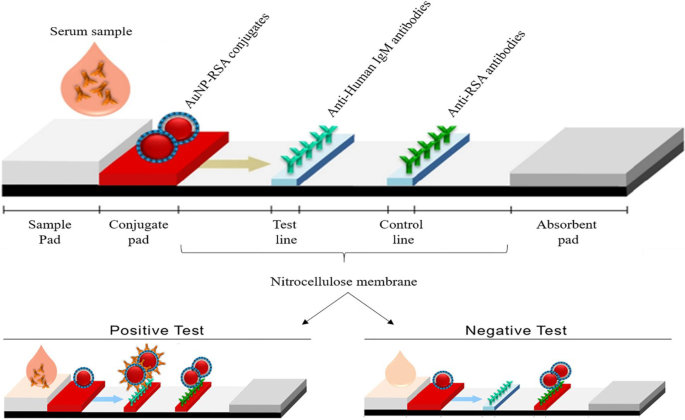
LFAs baseados em AuNP para detecção de IgM P. jirovecii anticorpos. A presença (teste positivo) ou ausência (controle negativo) de P. jirovecii os anticorpos podem ser diferenciados pela cor avermelhada do AuNP nas linhas teste e controle, ou apenas na linha controle, respectivamente. Reproduzido com permissão [70]. Copyright 2019, Frontiers in Microbiology
Um dos primeiros exemplos do uso de AuNPs como sonda de sinalização em um LFA foi para a detecção de células Ramos; o aptâmero TE02 foi usado como uma sonda de captura e o aptâmero TD05 como uma sonda de detecção. O biossensor aptamer-AuNP pode detectar visualmente um mínimo de 4.000 células Ramos sem qualquer instrumentação e 800 células Ramos com um leitor de fita portátil em 15 min. Usando este biossensor de detecção em sanduíche, o ensaio detectou com sucesso células Ramos enriquecidas com sangue humano [71] e foi usado como uma prova de conceito para o desenvolvimento de sistemas rápidos, sensíveis e de baixo custo para detecção qualitativa e quantitativa de células cancerígenas circulantes. Desde então, vários LFAs baseados em AuNP foram projetados para o diagnóstico de várias doenças infecciosas, incluindo doenças causadas por pneumonia por Pneumocystis [70], vírus Ebola [72], HIV, vírus da hepatite C e Mycobacterium tuberculosis [73] e mais recentemente o vírus SARS-CoV-2 [68].
Sistemas de imagem baseados em AuNP
AuNPs têm sido intensamente investigados para aplicações em bioimagem por causa de sua capacidade de absorver e espalhar luz combinando seus comprimentos de onda de ressonância, até 10 5 vezes mais do que os fluoróforos convencionais [74]. AuNPs têm um maior número atômico e densidade de elétrons (79 e 19,32 g / cm 3 ) em comparação com os agentes convencionais à base de iodo (53 e 4,9 g / cm 3 ), provando ser melhores agentes de contraste [24]. Os AuNPs se acumulam nas células ou tecidos doentes e induzem uma forte atenuação de raios-X, tornando o local alvo altamente distinto e facilmente detectável. AuNPs são anexados a frações químicas e agentes de bio-reconhecimento molecular que podem alvejar seletivamente antígenos específicos para induzir contraste distinto e específico para imagens de TC [75].
O sistema de imagem CT molecular direcionado in vitro foi obtido usando AuNPs funcionalizados com um aptâmero de RNA que se liga ao antígeno de membrana específico da próstata (PSMA). O conjugado de aptâmero AuNP-PSMA mostrou mais de quatro vezes a intensidade de CT para as células da próstata que expressam PSMA (LNCaP) em comparação com as células da próstata PC-3, que não possuem o receptor alvo [76]. Da mesma forma, o conjugado de aptâmero AuNP-ácido diatrizóico-AS1411 foi localizado em células CL1-5 (adenocarcinoma de pulmão humano) e camundongos com tumor CL1-5. O aptâmero AS1411 tem como alvo o receptor da nucleolina (NCL) que é expresso pelas células CL1-5 na superfície celular, enquanto o ácido diatrizóico é um agente de contraste à base de iodo. O conjugado AuNP-ácido diatrizóico-aptâmero AS1411 tinha uma curva de atenuação linear com uma inclinação de 0,027 mM unidade Au Hounsfield (HU −1 ) indicando o acúmulo de AuNPs no local do tumor [77]. Os AuNPs exibiram um tempo de retenção vascular mais longo, o que prolongou seu tempo de circulação no sangue [77,78,79] e melhorou o sinal de CT do ácido diatrizóico [77].
A Figura 5 mostra uma imagem de CT vascular in vivo de artérias coronárias usando AuNPs que foram conjugados à proteína de adesão de ligação de colágeno 35 (CNA35) para direcionar o colágeno I em infarto do miocárdio em roedores. O sinal AuNP ainda foi detectado no sangue 6 h após a administração intravenosa (i.v), que foi significativamente maior do que a meia-vida (5-10 min) dos agentes à base de iodo [79]. Esses efeitos foram replicados usando AuNPs verdes sintetizados com manana, que mostraram captação mediada por receptor e não toxicidade em células que expressam manose (DC 2.4 e RAW 264.7). Os AuNPs capeados com manana alvejaram seletivamente os nódulos linfáticos poplíteos in vivo após a injeção na pata traseira dos camundongos [38]. A imagem de TC baseada em AuNP pode fornecer informações significativas para o diagnóstico de várias doenças não limitadas à artéria coronária e cânceres [76, 77, 79,80,81]. O uso de AuNPs como agentes de contraste tem mostrado potencial em outros sistemas de imagem, como fotoacústica, imagem nuclear, ultrassom e ressonância magnética. Esses sistemas são extensivamente revisados em outro lugar [82, 83].
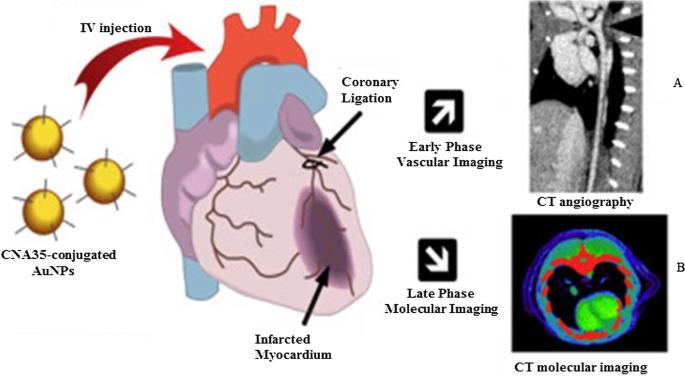
Imagens de TC in vivo usando AuNPs como agentes de contraste de TC. AuNPs capeados com manana e suas imagens de TC do linfonodo ( A ), e imagens de TC de AuNPs conjugadas com CNA35 de carga de cicatriz miocárdica ( B ) Reproduzido com permissão [79]. Copyright 2018, Elsevier
AuNPs em sistemas de detecção baseados em fluorescência
AuNPs são usados em sistemas de detecção baseados em fluorescência como agentes fluorescentes ou supressores fluorescentes. Em tamanhos ≤ 5 nm, AuNPs exibem propriedades de pontos quânticos (QDs) e podem ser usados em seu lugar. The Au 55 (PPh 3 ) 12 Cl 6 nanoclusters introduzidos em 1981 são provavelmente os mais intensamente estudados devido ao seu comportamento de tamanho quântico [7]. Desde então, vários AuNPs de tamanho quântico (AuNPsQ), como Au 25 (SR) 18 , Au 38 (SR) 24 e Au 144 (SR) 60 [84] foram estudados principalmente em detecção eletroquímica, pois são excelentes condutores eletrônicos e mediadores redox [85].
Eletrodos de filme AuNPsQ foram usados na fabricação de um imunossensor eletroquímico ultrassensível para a detecção do antígeno prostático específico (PSA). O imunossensor teve uma sensibilidade de 31,5 μA mL / ng e um limite de detecção de 0,5 pg / mL para PSA em 10 μL de soro humano não diluído. O imunoensaio teve um desempenho oito vezes melhor do que um imunossensor de floresta de nanotubos de carbono relatado anteriormente, contendo várias frações, na concentração de biomarcador que era mais baixa do que os níveis associados à presença de câncer. Como tal, pode ser usado para medir o biomarcador de teste em estados normais e doentes. O desempenho do imunossensor foi comparável ao método ELISA de referência [86]. AuNPsQ também foi incorporado em CaCO 3 de estrutura porosa esferas para formar um CaCO fluorescente 3 / AuNPsQ híbrido para a detecção de enolase neurônio-específica, um biomarcador diagnóstico e prognóstico para lesão cerebral traumática e câncer de pulmão. O sensor tinha um limite de detecção de 2,0 pg mL −1 [87]. Até agora, vários sistemas de detecção fluorescente baseados em AuNP foram relatados para a detecção de analitos associados com Hepatite B [73, 88], Influenza A [89], câncer [90] e lesão cardíaca [91].
AuNPs também são excelentes quenchers baseados em FRET [92]. Suas propriedades ópticas únicas (intensidade de sinal estável e resistência à fotodegradação), tamanho e capacidade de modificação os tornaram sondas atraentes em plataformas de detecção de fluorescência [93, 94]. AuNPs maiores (≥ 10–100 nm) têm baixos rendimentos quânticos que não são adequados para detecção direta de fluorescência; no entanto, sua capacidade de extinguir corantes fluorescentes sob um estado de energia de excitação relativamente alto os tornou inibidores de fotoluminescência eficazes [94]. Em princípio, as nanossondas de fluorescência são compostas por um fluoróforo doador (corante ou QDs) e um aceitador AuNPs e, quando colocado em estreita proximidade, a fluorescência do fluoróforo selecionado é extinta pelos AuNPs [94, 95]. Na ausência de um alvo, como indicado pela falta de um sinal fluorescente, a sonda de ácido nucleico hibridiza e forma uma estrutura em loop que traz o fluoróforo e um inibidor em suas extremidades opostas em estreita proximidade; enquanto a ligação do analito à sonda de ácido nucleico desloca o fluoróforo dos AuNPs, resultando em um sinal fluorescente [24, 94, 96]. Aproveitando as propriedades acima mencionadas, AuNPs foram incorporados em faróis moleculares para detecção in vitro (nanoesferas de ouro, AuNSs) e in vivo (nanobastões de ouro, AuNRs) da expressão de matriptase em células tumorais. Os dois faróis moleculares foram compostos de um local de clivagem de matriptase como um ligante entre os AuNPs e os fluoróforos. O farol molecular AuNS foi construído com o isotiocianato de fluoresceína (FITC), e o farol molecular AuNR tinha um corante fluorescente NIR (ácido mercaptopropiônico, MPA). Na ausência do alvo, os AuNSs e AuNRs, respectivamente, bloquearam a fluorescência de FITC e MPA. Cleavage of either FITC or MPA from the AuNP–molecular beacons in the presence of matriptase exhibited a quantifiable fluorescence signal. The fluorescent signal of the MPA–AuNR–beacon in the nude mice bearing HT-29 tumors lasted for 14 h in the tumor site, while the signal gradually disappeared from the non-tumor site over time [97].
The AuNPs were reported to have comparable or higher fluorescence quenching efficiency than organic quenchers such as 4-((4′-(dimethyl-amino)phenyl)azo)benzoic acid (DABCYL) [94, 98] and Black Hole Quencher-2 [99]. The fluorescence quenching efficiency of 1.4 nm AuNPs was compatible with the four commonly used organic fluorophores (FITC, rhodamine, texas red and Cy5). The fluorescence quenching efficiency of the AuNPs was similar to that of DABCYL, and unlike DABCYL, the AuNPs showed consistency in both low and high salt buffers [98]. In a competitive hybridization assay, 10 nm AuNPs showed superior (> 80%) fluorescence quenching efficiency for Cy3 dye than the commercial Black Hole Quencher-2 (~ 50%). The assay had a limit of detection of 3.8 pM and a detection range coverage from 3.8 pM to 10 nM for miRNA-205 in human serum, and it was able to discriminate between miRNAs with variations in their nucleotide sequence [99]. The competitive sensor arrays were not only sensitive [96, 99] but were able to differentiate between normal and diseased cells, as well as benign and metastatic cancers [96].
AuNP-Based Bio-barcoding Assay
AuNP-based bio-barcoding assay (BCA) technology has become one of the highly specific and ultrasensitive methods for detection of target proteins and nucleic acids up to 5 orders of magnitude than the conventional assays [100]. The assay relies on magnetic microparticle probes, which are functionalized with antibodies that bind to a specific target, and AuNP probes encoded with DNA that recognizes the specific protein target and antibodies. Upon interaction with the target DNA, a sandwich complex between the magnetic microparticle and AuNPs probes is formed. The sandwich is then separated by the magnet followed by thermal dehybridization to release the free bar-code DNA, enabling detection and quantification of the target [101, 102].
The AuNP-based BCA assay was able to detect HIV-1 p24 antigen at levels that was 100–150-fold higher than the conventional ELISA [103]. The detection limit of PSA using these systems was 330 fg/mL [104]. The versatility of AuNPs for the development of a BCA-based platform was further demonstrated by measuring the concentration of amyloid-beta-derived diffusible ligands (ADDLs), a potential Alzheimer's disease (AD) marker found in the cerebrospinal fluid (CSF). ADDL concentrations were consistently higher in the CSF taken from the subjects diagnosed with AD than in non-demented age-matched controls [105]. These results indicate that the universal labeling technology can be improved through the use of AuNPs to provide a rapid and sensitive testing platform for laboratory research and clinical diagnosis.
AuNP-Based Therapies
Metal-based drugs are not new to medicine; in fact, they are inspired by the existing metallic drugs used in clinical treatment of various diseases [9, 106,107,108,109]. The widely studied and clinically used metal-based drugs were derived from platinum (e.g., cisplatin, carboplatin, tetraplatin for treatment of advanced cancers), bismuth (for the treatment of infectious and gastrointestinal diseases), gold (for the treatment of arthritis) and gallium (for the treatment of cancer-related hypercalcemia) [108, 109]. The approval of cisplatin in 1978 by the FDA for the clinical treatment of cancer [107] further inspired research on other metals (such as palladium, ruthenium, rhodium) [32, 106, 110].
Owing to the bioactivities, which included anti-rheumatic, antibacterial and anticancer effects, and the biocompatibility of bulk gold [8, 9, 46, 111], AuNPs are extensively investigated for the treatment of several diseases. AuNPs displayed unique and novel properties that are superior to its bulk counterpart. AuNPs are highly stable and have a distinct SPR, which guides their application in medicine [112], as drug delivery and therapeutic agents. AuNPs have a lot of advantages over the conventional therapy; they have a longer shelf-life and can circulate long enough in the system to reach their targets [25] with [11, 49, 113] or without targeting molecules [14, 15, 24, 25, 114]. AuNPs can provide localized and selective therapeutic effects; some of the areas in which AuNPs were used in therapy are described below.
Therapeutic Effects of Untargeted AuNPs
The as-synthesized (i.e., unmodified or uncapped) AuNPs have been shown to have diverse therapeutic effects against a number of infectious [115, 116], metabolic and chronic diseases [3, 29, 50, 51]. Their antioxidant, anticancer, anti-angiogenic [3, 32], anti-inflammatory [3, 51] and weight loss [29, 50, 112] effects are beneficial for diseases such as cancer, rheumatoid arthritis, macular degeneration and obesity [5, 25, 113, 117]. The above-mentioned diseases are characterized by a leaky vasculature and highly vascularized blood vessels [5, 113], which provides the NPs an easy passage into the diseased tissues and increase the susceptibility of cells to their effects. Through the EPR effect, uncapped AuNPs can passively accumulate in the vasculature of diseased cells or tissues. Hence, AuNPs have been specifically designed to have anti-angiogenic effects in diseases where angiogenesis (the growth and extension of blood vessels from pre-existing blood vessels) spins out of control like cancer, rheumatoid arthritis, macular degeneration and obesity [5, 25, 113, 117]. Targeting and destroying the defective blood vessels prevent oxygen and nutrients from reaching the diseased cells, which results in their death. The pores in the blood vessels at the diseased site (especially in cancer and obesity) are 200–400 nm and can allow materials in this size range to pass from the vasculature into the diseased tissues and cells [14, 15, 25, 114].
The cellular uptake, localization, biodistribution, circulation and pharmacokinetics of the uncapped AuNPs rely strongly on size and shape [49]. Although these effects are applicable to all AuNPs, the biological effects of citrate-capped AuNPs (cAuNPs) are extensively studied and reviewed. Spherical cAuNPs demonstrated selective in vitro anticancer activity that was size and concentration dependent on murine and human cell lines [3, 51]. Different sizes (10, 20 and 30 nm) of cAuNPs showed differential effects in human cervical carcinoma (HeLa), murine fibroblasts (NIH3T3) and murine melanoma (B16F10) cells. The 20 and 30 nm cAuNPs showed a significant cell death in HeLa cells starting at the lowest concentration of 2.2 µg/mL, while the 10-nm NPs was toxic at concentrations ≥ 8.75 µg/mL. The activity of these NPs was negligible in the noncancerous NIH3T3 cells, especially the 10 and 20 nm. The 20 nm reduced viability by ≤ 5% at the highest concentration (35 µg/mL), and ~ 20% for the 10 and 30 nm. The IC50 values for 10, 20 and 30 nm cAuNPs in the Hela cells were 35, 2.2 and 4.4 μg/mL, respectively, while the IC50 values for noncancerous cells were higher than 35 µg/mL [3]. Using a concentration range of 0.002–2 nM, 13 nm cAuNPs induced apoptosis in rabbit articular chondrocytes and no effects were observed for 3 and 45 nm cAuNPs under the same conditions. The 13 nm cAuNPs induced mitochondrial damage and increased reactive oxygen species (ROS); these actions could not be blocked by pre-treatment with a ROS scavenger, the N-acetyl cysteine [51]. Size-dependent effects were also observed in vivo after injecting cAuNPs of various sizes (3, 5, 8, 12, 17, 37, 50 and 100 nm) into mice (8 mg/kg/week) for 4 weeks. The 8, 17, 12 and 37 nm were lethal to the mice and resulted in tissue damage and death after 14 days of treatment; the other sizes were not toxic and the mice survived the experimentation period. On the contrary, the same-size AuNPs at a concentrations up to 0.4 mM were not toxic to HeLa cells after 24 h exposure [118].
The cAuNPs can interact and accumulate nonspecifically within various tissues and organs in the body, especially in the reticuloendothelial system (RES) organs (blood, liver, spleen, lungs) [55, 119]. This was evident in high-fat (HF) diet-induced obese Wistar rats [55] and Sprague–Dawley rats [119] following acute (1 dose for 24 h) [55] and chronic (1 dose; 0.9, 9 and 90 µg/week over 7 week period) [119] exposure to 14 nm cAuNPs, respectively. Majority of the i.v injected cAuNPs were detected in the liver, spleen, pancreas, lungs, kidneys [55, 119] including the skeleton and carcass of the rats [119]. Chen et al. observed that after intraperitoneal (i.p) injection of a single dose (7.85 µg/g bodyweight) of 21 nm cAuNPs in lean C57BL/6 mice, they accumulated in the abdominal fat tissues and liver after 24–72 h [29], as well as the spleen, kidney, brain and heart in the HF-induced obese mice that were injected with the same dose daily for 9 weeks [50]. The cAuNPs reduced the abdominal WATs (retroperitoneal and mesenteric) mass and blood glucose levels 72 h post-injection [29]. In the diet-induced obese mice, the 21 nm cAuNPs demonstrated anti-inflammatory and anti-obesity effects [50]. They also improved glucose tolerance, enhanced the expression of inflammatory and metabolic markers in the retroperitoneal WATs and liver [50]. Both the 14 and 21 nm cAuNPs showed no sign of toxicity or changes in the markers associated with kidney and liver damage [29, 55, 119].
Similar findings were reported for plant-mediated AuNPs, without targeting molecules they can access, ablate tumors [40, 120] and obese WATs [121] in rodents. Differential uptake, distribution and activity of biogenic AuNPs also vary depending on the size and shape of the NPs. While certain sizes can pass through the vascular network and be retained at the site of the disease; others can be easily filtered out of the system through the RES organs and the mononuclear phagocytic system as shown in Fig. 6 [15, 114]. NPs can be removed by tissue-resident macrophages (TRMs) before they reach the disease cells. Those that escape the TRMs and do not reach the disease site, especially smaller NPs (≤ 5 nm), are excreted through glomerular filtration in the kidney [25, 114]. Pre-treatment with clodronate liposomes depleted the TRMs in the liver and spleen before exposure to 50, 100 and 200 nm AuNPs. This reduced uptake of the AuNPs by the liver, increased their half-life in the blood as well as their accumulation at the tumor site [122]. However, TRMs are not the only obstacle that the AuNPs that rely on EPR effect for uptake must overcome. EPR effect alone can only ascertain ≤ 1% AuNP uptake [15, 114], and depletion of the TRMs prior to treatment resulted in just ≤ 2% of NPs reaching the target [122]. The success of non-targeted AuNPs depends on their ability to reach and accumulate in the diseased tissues, of which passive targeting through the EPR effect might not be efficient. The NPs also need to circulate longer, escape early clearance, and most importantly show reduced bystander effects [25, 123]. These qualities can increase bioavailability and ensure selectivity and efficacy of the AuNPs. These can further be improved by changing the surface chemistry of the AuNPs as discussed below [15, 124].
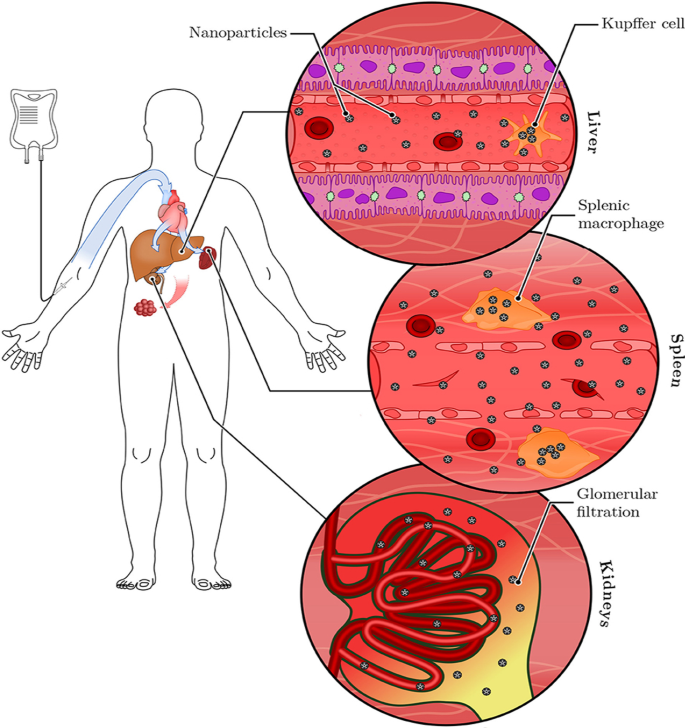
RES-based clearance of systemic administered AuNPs depends on their size. Large AuNPs accumulate in the liver, while smaller AuNPs are likely to end up in the spleen or be excreted in the urine via glomerular filtration. The AuNPs that escape the TRMs could accumulate in the diseased tissues. Reproduced with permission [114]. Copyright 2019, Frontiers in Bioengineering and Biotechnology
Therapeutic Effects of Surface-Functionalized AuNPs
The common strategy in AuNP-based therapeutics involves modifying the AuNP surface with therapeutic agents [3, 124,125,126]. The therapeutic agents can be drugs already used for the treatment of a particular disease or biomolecules with known inhibitory effects on cell signaling. In some instances, the therapeutic AuNPs have also been designed to have molecules that facilitate active targeting of the AuNPs toward specific cells and tissues. The molecules can easily adsorb on the AuNP surface by thiolation, chemical modification using chemistries such as 1-ethyl-3-(3-dimethylaminopropyl) carbodiimide (EDC), streptavidin/biotin binding [3, 124,125,126,127] and ionic interactions based on opposite charges between the NP surface and the biomolecules [124,125,126]. Functionalization of the AuNP surface influences their physicochemical properties and can affect their safety, biocompatibility and mobility. To ensure that the cargo carried by the AuNPs is delivered to the intended site, consideration should thus be given to both the physical and chemical properties of the AuNPs [124,125,126]. It is especially the size, shape, charge and the capping agents of the AuNPs that play an important role in the functionality of the AuNP conjugates [124] and can completely alter the pharmacokinetics of the AuNP-based therapeutics.
Functionalization allows for the development of customized nanosystems to reduce undesirable bystander effects often associated with traditional medicine. Functionalization of AuNPs can also prevent nonspecific adsorption of proteins onto the AuNP surface which can result in the formation a protein corona, resulting in the early clearance of the AuNPs through opsonization by the phagocytic cells [49, 123]. The surface charge of NPs can have a major influence on the behavior of NPs within biological environments. AuNPs with a neutral surface charge are unreactive and have a higher rate of escaping opsonization than charged AuNPs. Hydrophilic NPs will also behave differently to those with hydrophobic surfaces [49, 123]. PEG is one of the polymers most often used to mask AuNPs from phagocytic cells and has been shown to stabilize and enhance the biocompatibility of the AuNPs in numerous in vivo studies [49, 55, 123]. Pegylation improved the biocompatibility of 8.2 nm AuNPs by preventing neutrally and negatively charged AuNPs to bind to cell membranes or localize to any cellular components in African green monkey kidney (COS-1) cells [49]. And when the pegylated AuNPs were functionalized with a polyarginine cell penetrating moiety, the AuNPs were visualized on the cell membrane and inside the COS-1 cells [49]. Cell-penetrating peptides such as nuclear localization signal from SV40 virus, Tat from HIV and polyarginine peptides have been explored in translocation of AuNPs inside all cell type, normal or diseased. However, high specificity is required for clinical applications and can be achieved by taking advantage of the physiological differences between malignant and normal cells. This has been achieved by functionalizing the AuNPs with targeting molecules that recognize cell-specific receptors that are exclusively or overexpressed on the surface of target cells. This way, the AuNPs can be directed and delivered only to cells that express the target receptor. Therefore, conjugation of targeting moieties to the AuNPs (active targeting) will provide more selectivity, reduced bystander toxicity and enhanced efficacy since the AuNPs will be confined only to malignant tissues that express the target receptors [49, 55, 57, 113, 126, 127].
A good example to demonstrate the versatility of AuNPs is shown in Fig. 7, where four different molecules were conjugated onto the AuNPs to target two independent markers and mechanisms [11]. The multifunctional AuNPs were used for the treatment of leukemia (K562DR) cells that are resistant to doxorubicin (Dox). The 40 nm AuNPs were modified with two targeting moieties (folate and AS1411 aptamer) and two therapeutic agents (Dox and anti-miRNA molecules/anti-221). Folate molecule and AS1411 aptamer, respectively, recognize the folate and NCL receptors that are overexpressed on the cell surface and through receptor-mediated endocytosis will traffic the AuNP-conjugate into the cells. The AS1411 aptamer had dual functions, by also targeting the NCL receptor that is expressed inside the cells. After the AuNP-conjugate has been shuttled into the cells, the cargo (AS1411 aptamer, anti-221 and Dox) is off-loaded which independently act on three mechanisms that will synergistically bring about the demise of the cells. AS1411 aptamer together with anti-221 prevented leukemogenesis by suppressing the endogenous NCL and miR-221 function in the NCL/miR-221 pathway, thereby sensitizing the cells to the effects of Dox [11].
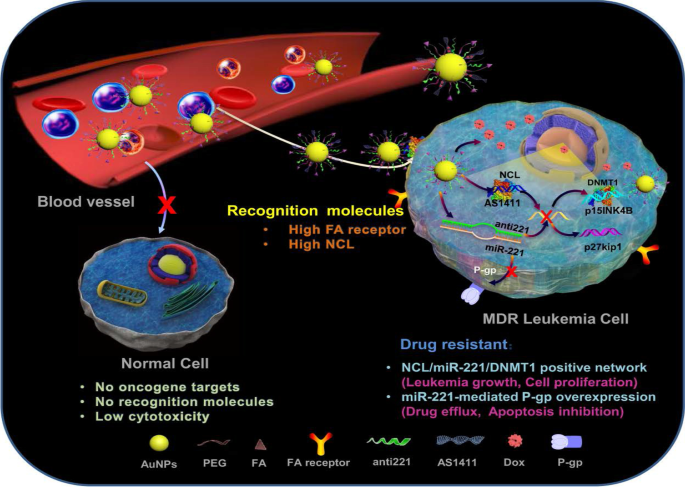
Multifunctional AuNPs in the treatment of multidrug-resistant (MDR) leukemia cells by increasing the sensitivity of the cells to Dox. Reproduced with permission [11]. Copyright 2019. Springer Nature. Folate (FA) receptor
Interestingly, similar dual targeting and treatment effects were achieved with green synthesized AuNPs without any additional molecules. With natural products acting as reducing agents, the biogenic AuNPs might also be more biocompatible than the chemically synthesized NPs [12, 40, 41, 43, 120]. MGF-AuNPs selectively targeted the laminin receptors in prostate (PC-3) and triple-negative breast cancer (MDA-MB-231) cells, and their xenografts in severe combined immunodeficiency (SCID) mice bearing these tumors [12, 40, 120]. In the normal SCID mice, the majority (85% at 30 min increasing to 95% after 24 h) of the i.v-injected MGF-AuNPs accumulated in the liver. Less than 10% were detected in the blood (2.7%), spleen (5%), lungs (0.6%), stomach, intestines and kidneys. When intra-tumorally injected in SCID mice-bearing prostate tumors, only 11% of the MGF-AuNPs were detected in the liver 24 h post-injection, while ~ 80% was in the tumor. Negligible amounts were found in the stomach, carcass and the small intestines. Some of the AuNPs were excreted through the renal and hepatic pathways in the urine and feces after 24 h [40, 120]. Nano Swarna Bhasma, a mixture consisting of AuNPs synthesized from mango peel extracts and phytochemicals from mango, turmeric, gooseberry and gum arabic, showed reduced toxicity toward normal endothelial cells after 48 h compared to the MDA-MB-231 cells [12].
Several studies have demonstrated that AuNPs have potential for clinical application. In combination with conventional drugs, it can be used to sensitize diseased cells to the drug effects [12, 128] and also prevent or reduce drug-related bystander effects [12]. AuNPs improved the pharmacokinetics of chemotherapeutic drugs, such as Dox [43, 129] and 5-fluorouracil (5-FU) [128]. Great improvements were mostly seen in the permeability and retention of drugs in the diseased cells, resulting in enhanced efficacy [130]. Dox-loaded AuNPs, which were non-toxic toward normal mouse fibroblast (L929) cells, also demonstrated selective toxicity toward fibrosarcoma tumors in mice [129]. 5-FU conjugated to the cAuNPs had better activity than 5-FU on its own in colorectal cancer cells [128]. AuNP co-treatment with chemotherapeutic drugs was highly efficient in improving the efficacy of chemotherapeutic drugs [12, 43, 128, 129, 131]. Orally ingested Nano Swarna Bhasma in combination with Dox and Cyclophosphamide reduced tumor volumes in SCID mice-bearing breast tumor cells and also showed acceptable safety profile and reduced bystander effects of the chemotherapeutic drugs in stage IIIA/B metastatic breast cancer patients [12]. Active targeting alone can ensure that the AuNPs are directly delivered into the desired targets, achieving a balance between efficacy and toxicity while minimizing damage to healthy tissues [14, 15, 49]. Controlled drug release is also among the many advantages offered by the AuNP-based systems and is crucial as it allows for localized and selective toxicity [49]. The AuNPs can be designed in such a way that their conjugates respond to internal (glutathione displacement, enzyme cleavable linkers, pH) or external (light, heat) stimuli to function [24, 25, 34, 128].
AuNPs as Transfection Agents in Gene Therapy
The use of AuNPs in gene therapy has shown promising outcomes by facilitating the delivery of genetic material to cells to silence or enhance expression of specific genes [24, 32, 132]. Thus, AuNPs can be used as transfection reagents in gene therapy for the treatment of cancer and other genetic disorders. AuNP conjugates have demonstrated higher transfection efficiency than experimental viral and non-viral gene-delivery vectors including polycationic reagents that has been approved for clinical use [24].
AuNPs are highly conductive and well suited for use as microelectrodes during electroporation for intracellular delivery of biomolecules for disease treatment. AuNPs significantly enhanced the performance of electroporation systems and have been used successfully for the delivery of DNA into hard-to-transfect cells such as the K562 cells [133]. To prevent cell loss which is often associated with electroporation, targeting moieties can be conjugated to the AuNPs to facilitate cellular uptake of AuNP conjugates through receptor-mediated mechanisms [133]. The use of AuNPs to transfect cells with oligonucleotide molecules also has the added advantage of increasing the half-life of these biomolecules and their efficacy [24, 32].
Untargeted AuNP conjugates are passively transported into cells and rely on the surface charge and AuNP shape for efficient transfection [24, 36, 134, 135]. The charge of the biomolecules that are conjugated onto AuNP surface plays a crucial role in their transfection efficiency; for instance, AuNPs functionalized with cationic molecules produce higher transfection efficiency than AuNPs functionalized with anionic molecules. Positively charged amino acids (lysine) can be attached on the NP surface to increase the rate of transfection. AuNSs [24] and AuNRs [36, 134, 135] are commonly used for transfections, and relative to the conventional transfection reagents (X-tremeGENE and siPORT), they inhibited the expression of target gene by > 70% in vitro [134] and in vivo [135]. In these studies, transfection efficiency was quantified based on target expression using RT-PCR and immunostaining [134, 135]. As transfection reagents, AuNPs provide long-lasting effects, localized gene delivery and higher efficacy [36, 134, 135]. Other types of nanomaterials (e.g., polymeric, liposomes, ceramic and carbon nanotubes) had received more attention for use in gene therapy than AuNPs. Six clinical trials using either polymeric or lipid-based nanomaterials for delivery of siRNA in solid tumors have been completed [36, 134, 136]. All of which suffer from low loading efficiency, low stability, and insufficient payload release [36, 136]. On the other hand, transfection systems based on AuNPs make use of easy chemistry that ensures efficient loading capacity and formation of stable complexes [36, 135]. Their safety can be controlled by manipulating their shape, size distribution and surface composition [36].
Antimicrobial Effects of AuNPs
MDR microbes are a major health concern and a leading cause of mortality, worldwide [21, 137,138,139,140,141]. These microorganisms have become resistant to conventional antimicrobial agents, due to over-prescription and misuse of these drugs [142]. No new antibiotics have been produced in over 40 years, mainly because the big pharmaceutical companies have retreated from their antibiotic research programs due to the lack of incentives [143]. As such, new and effective antimicrobial agents are urgently required to combat what could be the next pandemic, the antimicrobial resistance, and avoid surge in drug-resistant infections.
AuNPs are among the new generation of antimicrobial agents under review. They have shown broad antimicrobial (bactericidal, fungicidal and virucidal) effects against a number of pathogenic and MDR microorganisms and thus have potential to overcome microbial drug resistance [21, 142, 144]. Their antimicrobial effects are dependent on their physicochemical properties, especially their size, surface composition, charge and shape [21, 144]. Due to their small size, AuNPs can easily pass through the bacterial cell membrane, disrupt their physiological functions and induce cell death [35]. The exact antimicrobial mechanisms of AuNPs are not yet fully elucidated; despite this, some of the reported modes of actions that results from the interaction of various nanostructured materials (NSMs) with the bacterial cells are illustrated in Fig. 8. The highlighted mechanisms are also implicated in antimicrobial activity of AuNPs, they include induction of microbial death through membrane damage, generation of ROS and oxidative stress, organelle dysfunction, and alteration of gene expression and cell signaling [141].
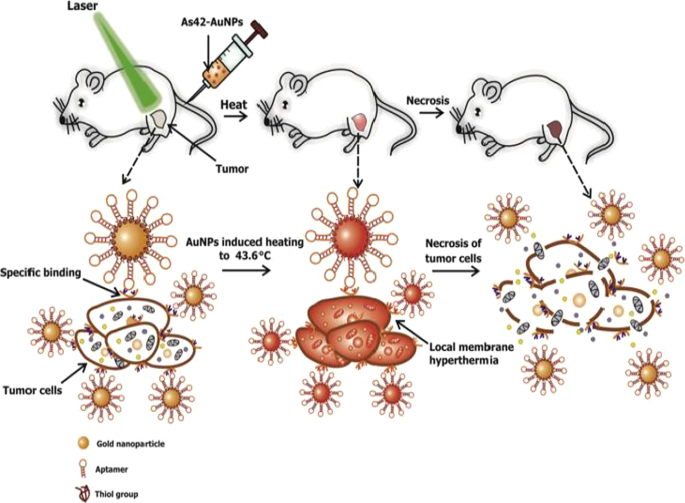
Antimicrobial mode of actions of the NSMs. Various NSMs can induce cell death by altering various biological functions, X represents alteration of cell signaling by de-phosphorylation of tyrosine residues in proteins as one of the mechanisms. Reproduced with permission [141]. Copyright 2018, Frontiers in Microbiology
AuNPs have multiple roles to play toward the development of antimicrobial agents, aside from being antimicrobial agents by themselves; they can serve as drug sensitizers and drug delivery vehicles [35, 58, 132, 145]. These features are applicable to both the chemical and green synthesized AuNPs, which have been reported to have antimicrobial effects against a number of human [21, 145,146,147] and waterborne [148] pathogenic strains. Generally, the test bacteria had shown low susceptibility toward the chemically synthesized AuNPs, i.e., the cAuNPs [21, 146, 147] and the NaBH4 -reduced AuNPs [149]. This was due to the repulsive forces between the negative charges on the AuNP surfaces and bacterial cells, thus preventing the interaction between AuNPs and the bacteria [21]. The activity of chemically synthesized AuNPs is based on their size, shape, concentration and exposure time. As an example, one study reported that NaBH4 -reduced AuNPs had no activity against Staphylococcus aureus (S. aureus ) and Escherichia coli (E. coli ) at 500 µg/mL for the duration of 6 h [149]. In contrast, another study showed a significant dose (1.35, 2.03 and 2.7 μg/mL) and size (6–34 nm vs 20–40 nm) dependent antibacterial effects of the NaBH4 -reduced AuNPs on Klebsiella pneumonia , E. coli , S. aureus and Bacillus subtilis [145].
The AuNPs are either used alone or in combination with other antimicrobial agents to treat microbial infections [35, 58, 132, 145]. When used in combination with other antimicrobial agents, the AuNP conjugates resulted in synergistic antimicrobial effects that surpassed the individual effects of the AuNPs and drugs [21, 35, 58, 132, 150]. These drugs were conjugated onto the AuNPs by either chemical methods [4, 151] or the drugs were used as reducing and capping agents [21, 149]. By so doing, the AuNPs improved drug delivery, uptake, sensitivity and efficacy. Some of the FDA-approved antibiotics and non-antibiotic drugs that were loaded onto the AuNPs are shown in Table 1 [4, 21, 149, 152]. Ciprofloxacin [152], cefaclor [149], lincomycin [4], kanamycin [21], vancomycin, ampicillin [151] and rifampicin [32] are among the antibiotics loaded on the AuNPs and demonstrated the versatility of AuNPs. These strategies were successful with various sizes and shapes of AuNPs, including gold silica nanoshells [152], AuNP-assembled rosette nanotubes [151] and AuNPs encapsulated in multi-block copolymers [153]. For instance, cefaclor-reduced AuNSs inhibited the growth of S. aureus and E. coli within 2–6 h depending on the concentration (10–50 µg/mL), while complete bacterial growth inhibition by the drug alone was only observed at 50 µg/mL after 6 h. The minimum inhibitory concentration (MIC) of the treatments was 10 µg/mL and 50 µg/mL for cefaclor-AuNPs and cefaclor, respectively [149].
AuNPs have presented properties that make them ideal candidates as alternative antimicrobial agents; the most important being their broad antimicrobial activity [21, 35, 58, 132, 150]. Owing to their biocompatibility and easily modifiable surface, microorganisms are less prone to developing resistance toward AuNPs [21]. For example, the kanamycin (Kan)-resistant bacteria (S . bovis , S . epidermidis , E . aerogenes , P . aeruginosa and Y . pestis ) showed increased susceptibility toward Kan-reduced AuNPs. The MIC values for Kan-AuNPs on the test bacteria were significantly reduced to < 10 µg/mL when compared to the MIC values for Kan alone at 50–512 µg/mL. This shows that AuNPs can restore the potency of antibiotics toward the drug-resistant strains by facilitating the uptake and delivery of the antimicrobial agents [21]. AuNPs can enhance drug-loading capacity and control the rate at which the drugs are released. AuNP hybrids with the multi-block copolymers increased the loading capacity of rifampicin and the drug’s half-life to 240 h. By sustaining the drug in the system for that long, ensured slow release of rifampicin from AuNPs at the target sites after oral administration of the AuNP conjugates to rats for 15 days. The drug on the surface was released within 24 h followed by the drug trapped in the polymer matrix after 100 h. And lastly, the drug entrapped between the AuNPs and the polymer matrix took over 240 h to be released in the interstitial space [153].
The AuNP hybrids also allow for the conjugation of multiple molecules with independent but synergistic functions. This was demonstrated by co-functionalization of the AuNPs with antimicrobial peptide (LL37) and the pcDNA that encode for pro-angiogenic factor (vascular endothelial growth factor, VEGF) and used in the treatment of MRSA-infected diabetic wounds in mice [132]. The AuNPs served dual functions, as a vehicle for the biomolecules, and also as transfection agent for the pcDNA. After topical application of the AuNP conjugates on the wound, the LL37 reduced MRSA colonies, while the pcDNA promoted wound healing by inducing angiogenesis through the expression of VEGF [132].
AuNPs have been shown to confer activity and repurpose some non-antibiotic drugs toward antimicrobial activity. The examples of repurposed drugs, which were used for the treatment of diseases other than bacterial infections, include 5FU [58], metformin [147] and 4,6-diamino-2-pyrimidinethiol (DAPT) [13, 112]. AuNPs as drug carriers are able to transport the drugs into the cells and allow direct contact with cellular organelles that resulted in their death [58, 147]. 5FU is an anti-leukemic drug, when attached to AuNPs was shown to kill some bacterial (Micrococcus luteus , S. aureus , P. aeruginosa , E. coli ) and fungal (Aspergillus fumigatus , Aspergillus niger ) strains [58]. While bacteria are resistant to DAPT, DAPT-AuNPs displayed differential antibacterial activity against the Gram-negative bacteria. Furthermore, conjugation of non-antibiotic drugs (e.g., guanidine, metformin, 1-(3-chlorophenyl)biguanide, chloroquine diphosphate, acetylcholine chloride, and melamine) as co-ligands with DAPT on AuNPs exerted non-selective antibacterial activity and a two–fourfold increased activity against Gram-negative bacteria [13]. When used in vivo, orally ingested DAPT-AuNPs showed better protection by increasing the intestinal microflora in E. coli -infected mice. After 4 weeks of treatment, the DAPT-AuNPs cleared the E. coli infection with no sign of mitochondrial damage, inflammation (increase in firmicutes ) or metabolic disorders (reduction in bacteroidetes ) in the mice [112].
The virucidal effects of the AuNP-based systems have been reported against several infectious diseases caused by influenza, measles [154], dengue [155, 156] and human immunodeficiency [115] viruses. Their anti-viral activity was attributed to the ability of AuNPs to either deliver anti-viral agents, or the ability to transform inactive molecules into virucidal agents [154, 156]. AuNPs synthesized using garlic water extracts inhibited measles viral growth in Vero cells infected with the measles virus. When the cells were exposed to both the virus and AuNPs at the same time, they blocked infection of Vero cells by the measles virus [154]. The AuNPs were nontoxic to the Vero cells up to a concentration of 100 µg/mL but inhibited viral uptake by 50% within 15–30 min at a concentration of 8.8 μg/mL [154]. Based on the Plaque Formation Unit assay, the viral load was reduced by 92% after 6 h exposure to 8.8 μg/mL of the AuNPs. The AuNPs interacted with the virus directly and blocked its transmission into the cells [154]. Modification of the AuNP surface with ligands that bind to the virus [156] or anti-viral agents [115, 155] protected them from degradation, enhanced their uptake and delivery onto the cells. The charge of the AuNPs also played a role, with cationic AuNPs being more effective in the delivery and efficacy of the AuNPs than the anionic and neutrally charged NPs. Cationic AuNPs complexed with siRNA inhibited dengue virus-2 replication in dengue virus-2-infected Vero and HepG-2 cells and also the virus infection following pre-treatment of the virus with AuNPs [155]. Inactive molecules are transformed into highly potent anti-viral agents after conjugation to AuNPs. One such example is the transformation of SDC-1721 peptide, a derivative of TAK-779, which is an antagonist of CCR5 and CXCR3 receptors for HIV-1 strain. SDC-1721 has no activity against the HIV-1, but when conjugated to the AuNPs it inhibited HIV-1 infection of the human phytohemagglutinin-stimulated peripheral blood mononuclear cells. The inhibitory effects of SDC-1721-AuNPs were comparable to the TAK-779 [115].
AuNPs as PT Agents
Diseased cells are sensitive to temperatures above 40 °C; cancer cells in particular appear to be even more sensitive to these high temperatures. Studies have shown that high fevers in cancer patients either reduced the symptoms of cancer or completely eradicated the tumors as a result of erysipelas infections [33, 157, 158]. Historically, fevers induced by bacterial infections, hot desert sand bath, or hot baths were used to increase the body temperature in order to kill the cancer cells [157]. These findings gave birth to PT therapy (PTT), which is mostly used for the treatment of cancer. PTT makes use of organic photosensitizers (indocyanine green, phthalocyanine, heptamethine cyanine) that are irradiated by the external source to generate heat energy that will increase the temperature to 40–45 °C (hyperthermia) in the target cells. Hyperthermia then triggers a chain of events (such as cell lysis, denaturation of the genetic materials and proteins), resulting in the destruction of the diseased cells [57, 158,159,160].
The organic dyes are used alone, or in combination with chemotherapy and radiotherapy for enhanced efficacy [157, 160]. Ideally, the effects of the PT agents must be confined to target cells and display minimal bystander effects. However, the organic PT dyes have several limitations such as toxic bystander effects, susceptibility to photobleaching and biodegradation [159]. In recent years, AuNPs are being explored as alternative PT agents as they exhibit strong plasmonic PT properties, and depending on their shape, they can absorb visible or NIR light. Absorption of light in the NIR spectrum is an added advantage that can allow deep tissue PTT [158, 161, 162]. Unlike organic dyes, AuNPs operate in an optical window where the absorption of light by interfering biological PT agents such as hemoglobin, melanin, cytochromes and water is very low [158, 161, 162].
The practicality of AuNP-based PTT has been demonstrated through in vitro and in vivo studies [158, 162, 163]. When the AuNPs are exposed to light, they can convert the absorbed light energy into thermal energy within picoseconds [57, 158, 159], consequently activating cell death via necrosis or apoptosis in the target cells or tissues. AuNP-based hyperthermia in diseased cells has been reported to occur at half the amount of the energy required to kill normal cells, thus perceived to be safer and better PT agents than the conventional dyes [33, 160]. AuNPs can be easily modified to have localized and enhanced PT activity by targeting and accumulating in only diseased cells through either active or passive targeting. And since the tumor environment is already hypoxic, acidic, nutrient starved and have leaky vasculature, the tumors will be most sensitive to the AuNP-based hyperthermia than the surrounding healthy cells and tissues [33, 160].
AuNP-based PTT has been extensively studied [158, 161, 162] and established that AuNPs (e.g., AuNRs, nanocages and nanoshells) that absorb light in the NIR spectrum are best for in vivo and deep tissue PTT [161]. While the ones that absorb and emit light in the visible spectrum (AuNSs and hollow AuNPs) have been demonstrated to treat diseases that affect shallow tissues (up to a depth of 1 mm), which could be of benefit to superficial tumors [158, 161, 162], ocular surgery [164, 165], focal therapy and vocal cord surgery [158, 165]. Although the PTT effects of AuNSs are limited in vivo or for use in deep tissues, combination therapy or active targeting can be incorporated to facilitate target-specific effects [158, 161, 163]. The AuNPs in the combination therapy will serve dual functions as both drug sensitizer and a PT agent, and was shown to enhance anticancer effects of chemotherapeutic drugs [158, 162, 163]. AuNS-Dox combination demonstrated enhanced cancer cell death after laser exposure when compared to the individual effects of the AuNSs and Dox with and without laser treatment [158].
Active targeting on its own can also improve AuNP uptake, localization and target-specific PT effects, which can be viewed in real time by adding fluorophores. AuNSs (25 nm) loaded with transferrin targeting molecules and FITC were shown to accumulate and destroy human breast cancer cells at a higher rate than in non-cancer cells and had better efficacy than the untargeted AuNSs [57]. An independent study also demonstrated that DNA aptamers (As42)-loaded AuNSs (As42-AuNP) induced selective necrosis in Ehrlich carcinoma cells that express HSPA8 protein, a receptor for the aptamers. None of these effects were observed in blood and liver cells mixed with target cells, or cells treated with the AuNSs without laser treatment [163]. The PT effects of the As42-AuNP were replicated in mice transplanted with Ehrlich carcinoma cells in their right leg. As shown in Fig. 9, tail-vein injections of As42-AuNPs followed by laser irradiation resulted in targeted PT destruction of the cancer cells. The As42-AuNPs reduced tumor size in a time-dependent manner; cell death was attributed to increased temperature up to 46 °C at the tumor site. The tumor in mice treated with As42-AuNPs without laser treatment and the AuNPs conjugated with nonspecific DNA oligonucleotide continued to grow but at the lower rate compared to mice injected with PBS. This suggests that the AuNPs were also localized in the tumor [163]. In cases where AuNSs are not efficient for deep tissue PTT, other shapes such as nanocages, nanoshells and AuNRs can be used [158]. Alternately, the visible light absorption of the AuNSs can be shifted to NIR by using processes such as two-photon excitation [57].
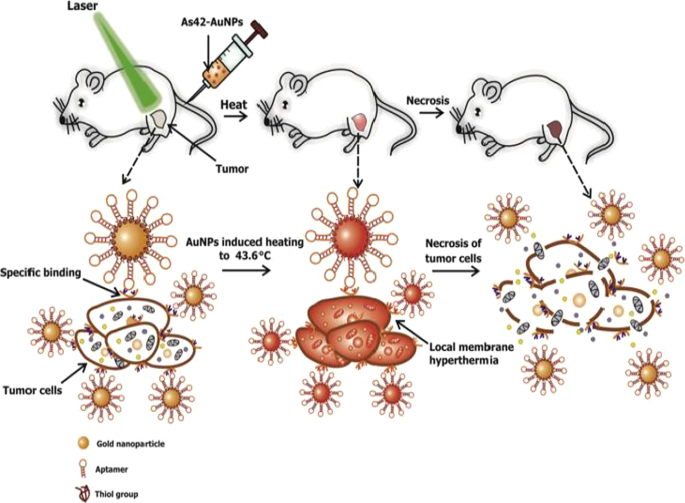
In vivo plasmonic PT therapy of cancer cells using targeted AuNSs. As42-AuNPs localized in HSPA8-expressing tumor cells after i.v injection. Exposure to laser treatment resulted in hyperthermia that caused cancer cell death. Reproduced with permission [163]. Copyright 2017, Elsevier
The PT effects of the AuNPs have also been reported for the reversal of obesity [52, 56], using hollow AuNSs (HAuNSs) [52] and AuNRs [56] for the PT lipolysis of the subcutaneous white adipose tissue (sWAT) in obese animals. The HAuNSs were modified with hyaluronate and adipocyte targeting peptide (ATP) to produce HA–HAuNS–ATP conjugate [52]. Hyaluronate was used to ensure topical entry of the HA–HAuNS–ATP through the skin [52, 166], while ATP will recognize and bind to prohibitin once the HAuNSs are internalized. Prohibitin is a receptor that is differentially expressed by the endothelial cells found in the WAT vasculature of obese subjects [5, 52, 55]. The HA–HAuNS–ATP was topically applied in the abdominal region of the obese mice, and through hyaluronate were transdermally shuttled through the epidermis into the dermis where the ATP located the sWATs (Fig. 10) . Illumination of the target site with the NIR laser selectively induced PT lipolysis of the sWAT in the obese mice and reduced their body weight [52]. The AuNRs were used in the photothermolysis-assisted liposuction of the sWATs in Yucatan mini pigs. The untargeted PEG-coated AuNRs (termed NanoLipo) were injected in the sWATs through an incision, followed by laser illumination to heat up the sWATs, which was then aspirated using liposuction. The amount of fat removed from NanoLipo-treated porcine was more than the one removed with conventional suction-assisted lipectomy (SAL). NanoLipo-assisted fat removal had several advantages over the conventional SAL; it took less time (4 min) for liposuction compared to 10 min for SAL, the swelling in the treated site healed faster, and the weight loss effects lasted over 3 months post-liposuction [56].
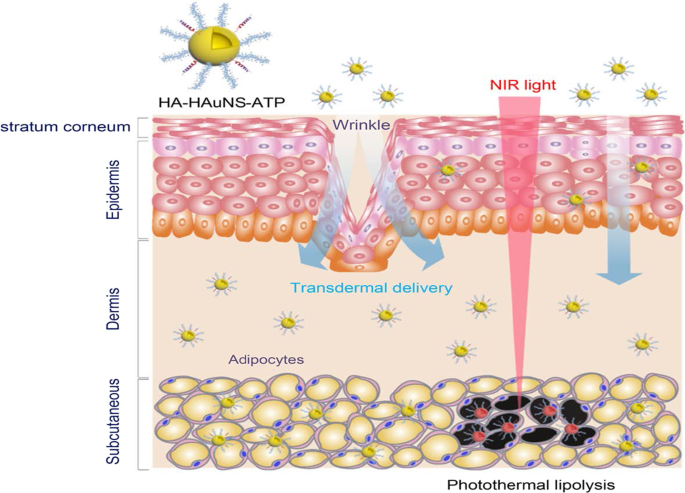
PT lipolysis of the sWATs using HA-HAuNS-ATP. The ATP was conjugated to the AuNSs for targeted delivery and destruction of the prohibitin-expressing sWATs after NIR laser exposure. Reproduced with permission [52]. Copyright 2017, American Chemical Society
AuNP-based PTT clearly offers a lot of advantages compared to the conventional agents. Their biocompatibility allows for broader applications both in vitro and in vivo. Moreover, they can be customized based on their shapes for shallow (AuNSs) [158, 161, 162] or deep tissue (AuNRs and stars) PTT [158, 161]. At 1–100 nm diameter, AuNPs and its conjugates can circulate long enough to reach and accumulate in the target tissues, with or without targeting moieties [159, 167]. Active targeting can be used to ensure localized PT effects through various routes of administration and might be effective for solid and systemic diseases. AuNP-based PTT can also be used to sensitize cancer cells when administered in combination with chemotherapy, gene therapy and immunotherapy [159]. Therefore, AuNP-based PTT has potential for treatment of chronic diseases [161].
Toxicity of AuNPs
AuNPs can play an important role in medicine, as demonstrated by the preclinical and clinical studies under review. Their full potential in clinical application as both diagnostic and therapeutic agents can only be realized if they do not pose any health and environmental hazards. While their use in vitro appears to be inconsequential, in vivo application can be hampered by their potential toxicity, which could be detrimental to human health. A major concern with their clinical use is that AuNPs are non-biodegradable and their fate in biological systems has not been fully studied [5, 30]. Although AuNPs are considered to be bio-inert and compatible, their properties (size, shape, charge and composition) raise concerns as they can alter their pharmacokinetics when used in biological environment [27, 34, 118]. The toxicity of AuNPs of varying sizes and shapes has been demonstrated in animals [27, 118]. These NPs can accumulate in the RES organs where they induce damage.
AuNPs are 1–100 nm in diameter which makes them smaller than most of the cellular components. At these sizes, AuNPs can passively transverse cellular barriers and blood vessels by taking advantage of the EPR effect in pathological cells. AuNPs with smaller diameters (1–2 nm) can easily penetrate cell membranes and biologically important cellular organelles such as mitochondria and nuclei [7, 168]. Accumulation of AuNPs in these organelles induces irreversible damage that can cause cellular demise. On the contrary, AuNPs larger than 15 nm are restricted to the cytoplasmic spaces and unable to penetrate internal organelles [168]. These features are desirable for targeting pathological cells, however, AuNPs can also be taken up by healthy cells and alter their physiology [118]. Administration of AuNP-based therapeutics can be done via different routes (i.e., intranasal, oral, transdermal, i.p or i.v) and transported through blood vessels into different tissues and organs [34, 118]. They are able to pass through the blood brain barrier and the placental barrier [34]. Toxicity is size dependent, with certain sizes of AuNPs being well tolerated, while others could be lethal to healthy tissues. Unfunctionalized AuNSs at 8, 17, 12, 37 nm caused physical changes (i.e., change the fur color, loss of bodyweight, camel-like back and crooked spine) within 14 days of treatment (2 doses of 8 mg/kg/week) in rats [118]. Most (> 50%) of the rats died within 21 days (i.e., after 3 doses), and abnormalities in the RES organs (liver, lungs and spleen) were observed. On the contrary, mice treated with 3, 5, 50 and 100 nm AuNPs were not affected by the NPs and no adverse effects or death occurred throughout the duration (50 days) of the study [118]. In diet-induced obese rats that received i.v injections of 14 nm cAuNPs, the NPs were detected in various tissues after 24 h and were mostly confined to the RES organs [55].
The shape, charge and surface chemistry of AuNPs can influence their toxicity. These factors can determine how AuNPs will interact with the biological systems, their cellular uptake and effects on the cells. AuNSs are readily taken up by cells and proven to be less toxic than other shapes such as rods and stars. AuNP surfaces are charged and will influence how they interact and behave within a biological environment [169]. Cationic AuNPs are likely to be more toxic compared to neutral and anionic AuNPs, as their charge allows these NPs to easily interact with negatively charged cell membranes and biomolecules such as DNA. Both the positively and negatively charged AuNPs have been associated with mitochondrial stress, which was not observed with the neutrally charged AuNPs [34, 35].
The shell that forms on the surface of the AuNP core can also influence the functioning of the NPs. These are usually reducing and/ or stabilizing agents such as citrate and CTAB, and once subjected to a biological environment, these molecules can cause either the desorption or absorption of biomolecules found in the biological environment. This can result in the formation of a corona or cause the NPs to become unstable. Citrate- and CTAB-capped AuNPs are highly reactive, which can facilitate the attachment of biocompatible polymers such as PEG, polyvinyl-pyrrolidone, poly (acrylic acid), poly(allylamine hydrochloride), and polyvinyl-alcohol) or biomolecules such as albumin and glutathione to prevent the formation of AuNP-corona with serum proteins. These molecules serve as a stabilizing agent and form a protective layer that can mask the AuNPs from attacks by phagocytes [7, 29, 34, 170] and prevent off-target toxicity [7]. As discussed in “AuNP-Based Therapies” section, AuNPs can be functionalized with targeting and therapeutic agents to define their targets and effects [34].
In addition to their physicochemical properties, the dosage, exposure time and environmental settings also influence the activity of AuNPs. Lower doses and short-term exposure times might render AuNP as nontoxic, while increasing these parameters will lead to cytotoxic effects [34]. Moreover, in vitro studies do not always simulate in vivo studies. At times, AuNPs that seem to be nontoxic in cell culture-based experiments end up being toxic in animal experiments. Many factors could be responsible for these discrepancies [118], and some steps have been identified that can guarantee the safety of AuNPs in biomedical applications. The biocompatibility and target specificity of AuNPs can be improved by modifying the surface of the NPs. Attaching targeting moieties on the AuNPs can channel and restrict their effects to specific targets or pathological cells [5, 55, 127]. Modification of AuNP surface with bio-active peptides provides a platform for developing multifunctional AuNPs with enhanced specificity, efficacy and potentially sustainable effects [11, 127]. All of these effects will be instrumental in the design and development of AuNP-based systems for clinical applications.
Clinical Application of AuNPs
Nanotechnology has the potential to shape the future of healthcare systems and their outcomes. Its promise of creating highly sensitive and effective nanosystems for medicine has been realized with the introduction of organic nanoformulations for cancer treatment. These systems have already paved the way for nanomaterials into clinical applications:doxil and abraxane have been in the market for over two decades and demonstrated the potential of nanotechnology in medicine [1, 2]. More recently, this technology has been used for the development of the SARS-CoV-2 lipid NP-based vaccine to fight against the COVID-19 pandemic [171]. Inorganic nanosystems such as AuNPs offer many advantages over their organic counterparts, yet few of these systems are used clinically (Table 2) [19, 32].
While several AuNP-based drugs are some of the inorganic nanomaterial-based drugs that were tested in clinical trials, they are not progressing at the same rate as organic liposome-based nanodrugs. Aurimune (CYT-6091) and aurolase were the first of AuNP-based formulations to undergo human clinical trials for the treatment of solid tumors. CYT-6091 clinical trials started in 2005 for delivery of recombinant TNF-α as an anticancer therapy in late-stage pancreatic, breast, colon, melanoma, sarcoma and lung cancer patients. CYT-6091 consists of 27-nm cAuNPs loaded with TNF-α and thiolated PEG. The CYT-6091 nanodrug has achieved safety and targeted biologic response at the tumor site at a dose lower than that required for TNF-α alone [16, 17]. CYT-6091 is approved and yet to start phase II clinical trials in combination with chemotherapy. Based on phase II clinical trial strategy, several variants of CYT-6091 have been developed and tested in preclinical studies. All the nanosystems contain TNF-α with either chemotherapy (paclitaxel, dox and gemcitabine), immunotherapy (Interferon gamma) or apoptosis inducing agents attached to the 27 nm cAuNPs [14,15,16]. The AuNP conjugates preferentially accumulated in the tumor sites after systemic administration through the EPR effect and vascular targeting effects of the TNF-α. The AuNPs were not detected in the healthy tissues, and the anti-tumor effects of TNF-α were restricted to the tumor environment [14, 16, 19].
The first clinical trial for the PT treatment with AuroLase® for refractory and/or recurrent head and neck cancers was completed. Information on the outcome of this trial is still pending. The second trial is set to evaluate the effects of AuroLase® on primary and/or metastatic lung tumors in patients where the airway is obstructed [19]. The number of human trials based on AuNP-based formulation is increasing, covering the treatment of a wide range of medical conditions including skin, oral, heart and neurological diseases. AuNP-formulation (150 nm silica-gold nanoshells coated with PEG), which is similar to AuroLase®, was approved for PT treatment of moderate-to-severe inflammatory acne vulgaris. The nanoshells were topically applied on the acne area and transdermally delivered into the follicles and sebaceous ducts through low-frequency ultrasound or massage. Nanoshells applied through massage were effective in penetrating the shallow skin infundibulum (90%) and the sebaceous gland (20%), while the low-frequency ultrasound can penetrate both shallow and deep skin tissues. NIR laser treatment resulted in focal thermolysis of the sebaceous glands in the affected area and disappearance of the acne [18, 167]. The gold–silica nanoshells were well-tolerated, showed no systemic toxic effects with minor side effects (reddiness and swelling) at the treatment site [18]. AuNPs offer many health benefits based on their unique properties but at the same time have raised a lot of political and ethical issues, and resulted in termination of some clinical studies (NCT01436123).
Conclusion and Future Perspectives
Applications of AuNPs in biomedicine are endorsed by their unique physicochemical properties and have shown great promise as theranostic agents. The increasing interest in biomedical applications of AuNPs is further encouraged by the biocompatibility and medical history of bulk gold, which suggests that the gold core in AuNPs will essentially display similar or improved properties [3]. But at the same time their small size can infer unique properties that will completely change their pharmacokinetics [144]. The diverse biomedical applications of AuNPs in diagnostics and therapeutics herein discussed demonstrate their potential to serve as adjunct theranostic agents. They can be used as drug delivery, PTT, diagnostic and molecular imaging agents [12, 33, 128]. In time, and with better knowledge of mechanisms of action, more AuNP-based systems will obtain approval for clinical use. However, the excitement of these biomedical applications of AuNPs should unequivocally be balanced with testing and validation of their safety in living systems before any clinical applications.
In conclusion, more work needs to be done to taper the toxicity of AuNPs. This can be achieved by introducing biocompatible molecules on their surface [14, 15, 58, 159], and developing new and better synthesis methods, such as the use of green chemistry to produce biogenic NPs. All these developments may further broaden the applications of AuNPs in nanomedicine. AuNPs are non-biodegradable, and off-target distribution could result in chronic and lethal effects. All these concerns must be addressed before clinical translation; the existing trials will soon provide some clarity on their impact in human health. Should their health benefits outweigh their potential risks as is the case with the existing clinical drugs, it is a matter of time before they are approved for clinical use.
Availability of Data and Materials
All the information in this paper was obtained from the studies that are already published and referenced accordingly.
Abreviações
- 5-FU:
-
5-Fluorouracil
- AD:
-
doença de Alzheimer
- ADDLs:
-
Amyloid-beta-derived diffusible ligands
- ASOs:
-
Antisense oligonucleotides
- AuNPs:
-
Gold nanoparticles
- AuNPsQ:
-
Quantum-sized AuNPs
- AuNRs:
-
Gold nanorods
- AuNSs:
-
Gold nanospheres
- BCA:
-
Bio-barcoding assay
- cAuNPs:
-
Citrate-capped AuNPs
- COVID-19:
-
Corona virus disease 2019
- CSF:
-
Cerebrospinal fluid
- CT:
-
Computed tomography
- CTAB:
-
Cetyltrimethylammonium bromide
- DABCYL:
-
4-((4′-(Dimethyl-amino)-phenyl)-azo)benzoic acid
- DAPT:
-
4,6-Diamino-2-pyrimidinethiol
- Dox:
-
Doxorrubicina
- EDC:
-
1-Ethyl-3-(3-dimethylaminopropyl) carbodiimide
- EPR:
-
Enhanced permeability and retention
- FA:
-
Folato
- FDA:
-
Food and Drug Administration
- FITC:
-
Fluorescein isothiocyanate
- FRET:
-
Fluorescence resonance energy transfer
- HAuNSs:
-
Hollow AuNSs
- HF:
-
High-fat
- HU −1 :
-
Hounsfield unit
- LFAs:
-
Lateral flow assays
- LSPR:
-
Localized surface plasmon resonance
- MDR:
-
Multidrug resistant
- MGF:
-
Mangiferin
- MPA:
-
Mercaptopropionic acid
- MWPLP:
-
Microwave-induced plasma-in-liquid process
- NCL:
-
Nucleolin
- NIR:
-
Near-infrared
- NSMs:
-
Nanostructured materials
- P. jirovecii :
-
Pneumocystis jirovecii
- PEG:
-
Polietileno glicol
- PSA:
-
Prostate-specific antigen
- PSMA:
-
Prostate-specific membrane antigen
- PT:
-
Photothermal
- PTT:
-
Photothermal therapy
- QDs:
-
Quantum dots
- RES:
-
Reticuloendothelial system
- ROS:
-
Reactive oxygen species
- SARS-CoV-2:
-
Severe acute respiratory syndrome-coronavirus-2
- SCID:
-
Severe combined immunodeficiency
- SPR:
-
Surface plasmon resonance
- sWAT:
-
Subcutaneous white adipose tissue
- TOAB:
-
Tetrabutylammonium bromide
- TRMs:
-
Tissue-resident macrophages
- VEGF:
-
Vascular endothelial growth factor
- WAT:
-
White adipose tissue
Nanomateriais
- Nanopartículas de ouro para sensores quimio
- Nanopartículas para terapia do câncer:progresso e desafios atuais
- Avanços e desafios dos nanomateriais fluorescentes para aplicações biomédicas e de síntese
- Compostos de grafeno e polímero para aplicações de supercapacitor:uma revisão
- A preparação da nanoestrutura de casca de gema de Au @ TiO2 e suas aplicações para degradação e detecção de azul de metileno
- Poliglicerol hiper-ramificado modificado como dispersante para controle de tamanho e estabilização de nanopartículas de ouro em hidrocarbonetos
- Preparação de micrromateriais híbridos MnO2 revestidos com PPy e seu desempenho cíclico aprimorado como ânodo para baterias de íon-lítio
- Fabricação, caracterização e citotoxicidade de nanopartículas de carbonato de cálcio derivadas de casca de ouro conjugada em forma esférica para aplicações biomédicas
- Avanços recentes em métodos sintéticos e aplicações de nanoestruturas de prata
- Platycodon saponins from Platycodi Radix (Platycodon grandiflorum) para a síntese verde de nanopartículas de ouro e prata